BCR/ABL can promote CD19+ cell growth but not render them long-term stemness
Introduction
B-cell acute lymphoblastic leukemia (B-ALL) is a clonal malignant disease caused by various genetic lesions in hematopoietic cells and is characterized by an accumulation of immature B-cell precursors leading to the suppression of normal hematopoiesis (1-3). Chromosomal translocations are defining characteristics of B-ALL, such as TEL/AML1, MLL rearrangements, BCR/ABL and E2A/PBX1 (4).
BCR/ABL, the first fusion oncogene to be found in hematological malignancies, is generated by the t(9;22)(q34;q11) chromosomal translocation, also known as Philadelphia chromosome (Ph). The fusion gene encodes a constitutively active tyrosine kinase, which alters signaling pathway, subverts the controls of normal proliferation, blocks differentiation and promotes resistance to apoptosis (5,6). The fusion protein was found in all of the human chronic myelogenous leukemia (CML) (6) and in part of B-ALL. The frequency of BCR/ABL in B-ALL patients shows a positive correlation with age accounts for only 5% in patients with B-ALL aged <20 years, while escalates to 49% in patients aged >40 years (7,8). According to the difference of break point in BCR (break cluster region), there are three BCR/ABL fusion proteins with different sizes: p190, p210 and p230 (6). The BCR/ABL p190 fusion gene accounts for 90% in pediatric Ph+ B-ALL and 50% to 80% in adult Ph+ B-ALL; BCR/ABL p210 is the major form of CML, while the p230 BCR/ABL mutation is rarely associated with Ph+ chronic neutrophilic leukemia.
While significant advances have been made in the treatment of CML in chronic phase by targeting the kinase activity of BCR/ABL (9) [5-year event-free survival and progression-free survival rate is 83% and 93%, respectively (10)], imatinib, the first generation BCR/ABL tyrosine kinase inhibitor, is not effective in treating patients with Ph+ B-ALL. Dasatinib, a dual ABL and SRC kinase inhibitor, works reasonably well in Ph+ B-ALL (11) due to SRC inhibition. However, the remission time is still relatively short due to its inability to eradicate leukemia stem cells (12).
Cancer is comprised of a group of heterogeneous malignant cells with hierarchical organization, and this hierarchical structure is composed of a mixture of immature cells and more differentiated cells. Cells capable of self-renewal and reconstituting the entire tumor are termed cancer stem cells. Understanding how cancer acquires stemness is important to develop effective therapies. Acute myeloid leukemia (AML) is the first hematologic malignancy proven to contain leukemic stem cells (LSCs). The CD34+CD38− subpopulation of human AML cells can reconstruct AML in NOD/SCID mice (13,14). Subsequent research demonstrated that driver mutations could transform normal hematopoietic stem cells (HSCs) into pre-leukemic HSC cells, which could evolve into unregulated AML stem cells with the accumulation of subsequent mutations (15). However, progenitor cells can also acquire stemness after transformation by certain oncogenes in AML. For example, granulocyte progenitor cells (GMPs) can be converted into AML stem cells via overexpression of MLL/AF9 fusion gene (16), and MOZ/TIF2 can also transform myeloid progenitor cells (CMPs and GMPs) into AML stem cells (17). But unlike AML, stem cells in the chronic phase of CML only derive from HSCs, as BCR/ABL cannot confer stemness on progenitor cells (17). However, in B-ALL, the origin of LSCs remains unclear (12,18,19).
In this study, we investigated whether the committed B-lymphoid cells can be conferred to LSCs by BCR/ABL. We found that transducing BCR/ABL in CD19+ cells (committed B-lymphoid cells including pro-B, pre-B, immature and mature B cells) can promote their growth in vitro and induce B-ALL like disease in vivo. However, only BCR/ABL transduced bone marrow (BM) cells can be transplanted multiple times in recipient mice, and the frequency of long-term LSCs ranges from 1/135 to 1/629. These studies suggest that BCR/ABL is unable to confer the long-term stemness to committed B-lymphoid progenitors.
Methods
Cell culture
The BM cells were cultivated in RPMI-DMEM (GIBCO, CA, USA) supplemented with 10% Fetal bovine serum (FBS, MOREGATE, AUS), and 10 ng/mL Interleukin-7 (IL-7, R&D, MN, USA). Bosc23 cells were cultured in DMEM containing 10% FBS. NIH3T3 cells were maintained in DMEM supplemented with 10% bovine serum (BS, MOREGATE, AUS).
Isolation of cells
CD19+ cells were selected by the Magnetic cell isolation and cell separation (MACS) technology (Miltenyi Biotec, GER). Briefly, all cells were prepared using 30 µm nylon mesh. Cells were then positively selected by the CD19 antibody conjugated beads (MACS CD19 Microbeads, Miltenyi Biotec, GER), with MACS separators. Isolation by MACS resulted in more than 95% purity as assessed by flow cytometry.
Colony forming assay
The BM cells were isolated from wide type BALB/cByJ mice and were infected with BCR/ABL or vector control retroviruses for 6 h, as previously described (20). Totally 4×105 infected BM cells or 1.2×105 infected CD19+ cells were plated in MethoCult M3630 methylcellulose medium (StemCell Technologies, Vancouver, Canada). The colonies were analyzed by fluorescence microscope on day 7.
Flow cytometry analysis
Cells isolated from peripheral blood or BM were treated with red blood cell lysis buffer, and single-cell suspensions were made for immunostaining analysis. The 10 µL peripheral blood were stained with each of the following antibodies. The antibodies used in the analysis were: Gr-1-PE, Mac-1-PE-cy7, B220-APC-cy7, CD19-APC, CD43(S7)-Percp-cy5.5, CD24-PE, BP-1-BV421, IgM-PE (BD, San Diego, CA). Flow cytometry experiments were performed on the fluorescence-activated cell sorting (FACS) machine (LSR II system, BD Biosciences, San Jose, CA) and data was analyzed using FlowJo, 7.6.1 software (Tree Star, San Carlos, CA).
Retrovirus production, and hematopathological analysis
Retroviruses were produced and normalized to equivalent titers for mouse B-ALL model as previously described (20). Viral titer was calculated in transducing units (TU) by multiplying the percentage of NIH3T3 cells expressing GFP in the total number of cells on the dish. BALB/cByJ mice (Shanghai SLAC Laboratory Animal Co.Ltd, Shanghai, China) were used for all experiments. The 10 µL peripheral blood cell from each mouse was obtained and used for WBC counts or flow cytometry analysis.
All procedures were conducted with the ethical approval of the Animal Care & Welfare Committee of Shanghai Jiao Tong University School of Medicine.
BM transduction and transplantation
To establish B-ALL, BM cells from donor mice were infected with BCR/ABL retrovirus for 6 h as previously described (20). Immediately after transduction, 1×106 whole BM cells or 3×105 CD19+ cells with 2×105 helper cells (BM cells from syngeneic mice) were transplanted into lethally irradiated (2×350 cGy) syngeneic female recipient mice. In the secondary or trinary transplantation, the GFP+ cells (leukemic cells) were isolated from B-ALL mice of primary or secondary transplantations by a MoFlo high-speed cell sorter (Dako-Cytomation, Carpinteria, CA). Then 1×105 GFP+ cells were transplanted into semi-lethally irradiated (1×350 cGy) syngeneic recipient female mice. All recipient mice were checked routinely as previously described (21).
Limited dilution assay
We isolated GFP+ cells from BM of B-ALL mice induced by BCR/ABL using FACS and transplanted them into semi-lethally irradiated (1×350 cGy) recipient mice with a number of 10, 100, 1,000, 10,000, 100,000 respectively. According to the survival rate of each group of mice that received differential number of leukemic cells, we calculated the frequency of leukemic stem cells in B-ALL induced by BCR/ABL [using the L-Calc software (v1.1, StemCell Technologies)].
Statistical analysis
Graphpad Prism 5 software was used for statistical data analysis. The Kaplan-Meier survival curve was used for survival analysis. Statistical comparisons of the results between groups were analyzed using the Student’s t-test method. *, P<0.01; **, P<0.001; ***, P<0.0001 was considered significant statistically.
Results
BCR/ABL promotes CD19+ B-lymphoid cells growth in vitro
To check whether BCR/ABL+ B-ALL could be initiated from committed B-lineage cells, we first examined the ability of BCR/ABL to stimulate CD19+ cells in forming colonies in vitro. We infected the BM cells with retrovirus containing BCR/ABL fusion oncogene for 6 h, then plated 4×105 infected BM cells or 1.2×105 CD19+ cells sorted from BCR/ABL infected BM cells into methylcellulose medium that supports the optimal growth of mouse pre-B cells (MethoCultTMM3630). BM cells and CD19+ cells infected with vector retrovirus were included as negative controls. Colonies were scored after 7 days culture. Both the size and number of B-lymphoid colonies are significantly increased in the BCR/ABL-transducing group comparing to that of the control (Figure 1A,B). This result demonstrates that BCR/ABL can promote growth of B-lineage committed cells.
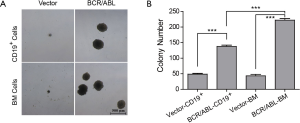
Transducing CD19+ cells with BCR/ABL can induce B-ALL like disease in mice
Since BCR/ABL can promote the colony formation of CD19+ cells in vitro, we examined whether BCR/ABL-expressing CD19+ cells could induce B-ALL in vivo. We first infected the mouse BM cells with retroviruses containing the BCR/ABL fusion oncogene for 6 h. We then transplanted either sorted CD19+ cells or unsorted BM cells that contain equal number of CD19+ cells into lethally irradiated syngeneic recipient mice. Both BCR/ABL-CD19+ and BCR/ABL-BM recipient mouse groups succumbed to a lethal disease, with no significant difference in survival time (Figure 2A). We analyzed the peripheral blood of the two groups of mice during the course of this experiment by flow cytometer and found that there is no significant difference in the amount of GFP+ leukemia cells (data not shown). Immunophenotyping analysis shows that the GFP+ cells from both groups of mice are CD19+B220+CD43+IgM− (Figure 2B), a typical phenotype of B-ALL. These results demonstrate that expression of BCR/ABL in both the whole BM cells and CD19+ cells can efficiently induce B-ALL like disease in mice.
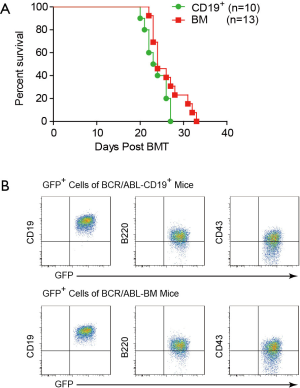
B-ALL developed from BCR/ABL expressing CD19+ cells cannot be serially transplanted in recipient mice
To investigate whether BCR/ABL could transform CD19+ cells into leukemia stem cells, we carried out BM serial transplantation experiments (Figure 3A). First, we isolated GFP+ cells from the primary BCR/ABL-CD19+ mice or BCR/ABL-BM mice two weeks after the BM transplantation and transplanted them into secondary recipient mice. We found that though both groups of mice developed B-ALL like disease, BCR/ABL-CD19+ secondary recipient mice had a significantly longer latent period of time than that of BCR/ABL-BM secondary recipient mice (Figure 3B). Consistently, expansion of GFP+ cells in BCR/ABL-CD19+ secondary mice was significantly slower (Figure 3C). The decrease of GFP+ cells in the peripheral blood of BCR/ABL-BM secondary mice coincides with the development of pleural effusion in these mice (Figure 3C and data not shown).
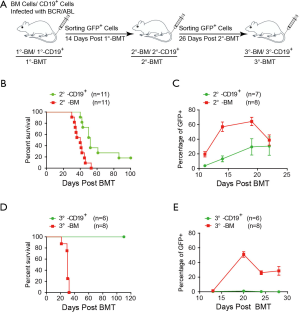
We then performed the tertiary transplantation experiment using the leukemic cells isolated from BCR/ABL-CD19+ secondary mice or BCR/ABL-BM secondary mice. Interestingly, while all BCR/ABL-BM tertiary mice succumbed to B-ALL like disease within 31 days post BM transplantation, none of the BCR/ABL-CD19+ tertiary mice developed signs of the disease during the course of the experiment (Figure 3D). Consistently, GFP+ cells were not expanded in BCR/ABL-CD19+ tertiary mice (Figure 3E).
These results demonstrate that although BCR/ABL-expressing CD19+ cells are able to induce B-ALL in the primary mice, they cannot be serially transplanted into recipient mice as those of BCR/ABL-BM cells. The results indicate that BCR/ABL is unable to transform committed B lymphoid cells into leukemia stem cells, implying that BCR/ABL B-ALL stem cells are originated from CD19−BM cells.
Leukemia stem cell frequency in B-ALL mice induced by BCR/ABL
As described above, BCR/ABL-BM mice contains B-ALL stem cells. To measure the frequency of leukemia stem cells, we performed a limiting dilution experiment. In this experiment, the recipient mice were transplanted with 10, 100, 1,000, 10,000, 100,000 GFP+ cells, respectively, isolated from BCR/ABL-BM secondary mice. Six months later, all the mice that receiving more than 1,000 cells were succumbed to B-ALL, while 82%, 18% and 0% mice receiving 1,000 cells, 100 cells and 10 cells developed B-ALL, respectively (Figure 4). This result shows that the frequency of leukemia stem cells is between 1/135 to 1/629 with a 95% confidence interval.
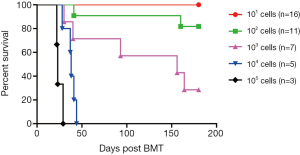
Discussion
In this study, we found that expressing BCR/ABL in CD19+ cells can stimulate B-lymphoid colony formation and induces B-ALL like disease in mice. However, the B-ALL is incapable of serial transplantation in recipient mice as the B-ALL developed from BCR/ABL transduced BM cells. The results indicate that BCR/ABL is unable to transform committed B lymphoid cells into leukemia stem cells, implying that BCR/ABL B-ALL stem cells are originated from CD19− BM cells.
The identity of leukemia stem cells in BCR/ABL+ B-ALL remains elusive. In patients, BCR/ABL+ B-ALL CD34+CD38− or CD34+CD38−CD58− (22) as well as CD34+CD38−CD19+ (18) subpopulation have been considered leukemia stem cells. In mice, leukemic cells with CD19+B220+CD43+ (12) immunophenotype were shown to resist the treatment of dasatinib and were considered as B-ALL stem cells. Though we cannot rule out the possibility that the LSCs could differentiate into CD19+ cells and maintain stemness, our studies imply that LSCs in BCR/ABL+ B-ALL originate from CD19− hematopoietic stem/progenitor cells. In addition, we show here that the frequency of stem cells of BCR/ABL induced B-ALL is between 1/135 to 1/629, unlikely to be the major population of the CD19+B220+CD43+ leukemia cells. Our and others’ previous work have shown that expressing BCR/ABL in HSCs induces CML in mouse (23,24), suggesting that BCR/ABL+ B-ALL stem cells are not derived from HSCs either. The identity and roles of the BCR/ABL+ B-ALL stem cells in the development of B-ALL and relapse of the disease need to be further investigated.
CD19 chimeric antigen receptor (CAR) modified T cells is a new therapeutic method for B-lineage hematopoietic malignancies (25-27) and have obtained a 60–80% response rate in patients with relapsed or refractory B-ALL (28). In this approach, T cells are genetically modified ex vivo to express a CAR with the CD19 antigen recognition sequence of a monoclonal antibody linked to intracellular T-cell signaling domains (29,30). Modified T cells can recognize and kill the leukemic cells expressing the CD19 antigen. CD19 is an excellent target for CAR-T therapy because it is highly expressed on more than 95% B-lineage hematopoietic malignancies (28). However, relapse of the diseases after CD19 CAR-T cell therapy remains a challenge (31). This clinical problem may also be caused by the residual of LSCs after therapy. Whether CD19 is expressed in B-ALL LSCs is a key determinant for CD19 CAR-T therapy. Our finding that BCR/ABL is unable to confer the long-term stemness to committed B-lymphoid progenitors (CD19+ cells) suggests that CD19 CAR-T cell therapy may not be effective in eradicating LSCs in BCR/ABL+ B-ALL.
Acknowledgements
We thank Huanbin Zhao, Qingsong Qiu, Min Wu and Chun Zhang for technical assistance.
Funding: This work was supported by the National Key Research and Development Program (2016YFC0902800) and the National Natural Science Foundation of China (81230055 and 81530006 to R Ren).
Footnote
Conflicts of Interest: The authors have no conflicts of interest to declare.
Ethical Statement: All procedures were conducted with the ethical approval of the Animal Care & Welfare Committee of Shanghai Jiao Tong University School of Medicine.
References
- Inaba H, Greaves M, Mullighan CG. Acute lymphoblastic leukaemia. Lancet 2013;381:1943-55. [Crossref] [PubMed]
- Abraham SA, Hopcroft LE, Carrick E, et al. Dual targeting of p53 and c-MYC selectively eliminates leukaemic stem cells. Nature 2016;534:341-6. [Crossref] [PubMed]
- Loghavi S, Kutok JL, Jorgensen JL. B-acute lymphoblastic leukemia/lymphoblastic lymphoma. Am J Clin Pathol 2015;144:393-410. [Crossref] [PubMed]
- Roberts KG, Mullighan CG. Genomics in acute lymphoblastic leukaemia: insights and treatment implications. Nat Rev Clin Oncol 2015;12:344-57. [Crossref] [PubMed]
- Lu R, Wang P, Parton T, et al. Epigenetic Perturbations by Arg882-Mutated DNMT3A Potentiate Aberrant Stem Cell Gene-Expression Program and Acute Leukemia Development. Cancer Cell 2016;30:92-107. [Crossref] [PubMed]
- Melo JV. The diversity of BCR-ABL fusion proteins and their relationship to leukemia phenotype. Blood 1996;88:2375-84. [PubMed]
- Zhao H, Liu P, Zhang R, et al. Roles of palmitoylation and the KIKK membrane-targeting motif in leukemogenesis by oncogenic KRAS4A. J Hematol Oncol 2015;8:132. [Crossref] [PubMed]
- Moorman AV, Harrison CJ, Buck GA, et al. Karyotype is an independent prognostic factor in adult acute lymphoblastic leukemia (ALL): analysis of cytogenetic data from patients treated on the Medical Research Council (MRC) UKALLXII/Eastern Cooperative Oncology Group (ECOG) 2993 trial. Blood 2007;109:3189-97. [Crossref] [PubMed]
- Keam SJ. Dasatinib: in chronic myeloid leukemia and Philadelphia chromosome-positive acute lymphoblastic leukemia. BioDrugs 2008;22:59-69. [Crossref] [PubMed]
- Papaemmanuil E, Gerstung M, Bullinger L, et al. Genomic Classification and Prognosis in Acute Myeloid Leukemia. N Engl J Med 2016;374:2209-21. [Crossref] [PubMed]
- McCormack PL, Keam SJ. Dasatinib: a review of its use in the treatment of chronic myeloid leukaemia and Philadelphia chromosome-positive acute lymphoblastic leukaemia. Drugs 2011;71:1771-95. [Crossref] [PubMed]
- Hu Y, Swerdlow S, Duffy TM, et al. Targeting multiple kinase pathways in leukemic progenitors and stem cells is essential for improved treatment of Ph+ leukemia in mice. Proc Natl Acad Sci U S A 2006;103:16870-5. [Crossref] [PubMed]
- Bonnet D, Dick JE. Human acute myeloid leukemia is organized as a hierarchy that originates from a primitive hematopoietic cell. Nat Med 1997;3:730-7. [Crossref] [PubMed]
- Lapidot T, Sirard C, Vormoor J, et al. A cell initiating human acute myeloid leukaemia after transplantation into SCID mice. Nature 1994;367:645-8. [Crossref] [PubMed]
- Shlush LI, Zandi S, Mitchell A, et al. Identification of pre-leukaemic haematopoietic stem cells in acute leukaemia. Nature 2014;506:328-33. [Crossref] [PubMed]
- Krivtsov AV, Twomey D, Feng Z, et al. Transformation from committed progenitor to leukaemia stem cell initiated by MLL-AF9. Nature 2006;442:818-22. [Crossref] [PubMed]
- Huntly BJ, Shigematsu H, Deguchi K, et al. MOZ-TIF2, but not BCR-ABL, confers properties of leukemic stem cells to committed murine hematopoietic progenitors. Cancer Cell 2004;6:587-96. [Crossref] [PubMed]
- Castor A, Nilsson L, Astrand-Grundström I, et al. Distinct patterns of hematopoietic stem cell involvement in acute lymphoblastic leukemia. Nat Med 2005;11:630-7. [Crossref] [PubMed]
- Kong Y, Yoshida S, Saito Y, et al. CD34+CD38+CD19+ as well as CD34+CD38-CD19+ cells are leukemia-initiating cells with self-renewal capacity in human B-precursor ALL. Leukemia 2008;22:1207-13. [Crossref] [PubMed]
- Roumiantsev S, de Aos IE, Varticovski L, et al. The src homology 2 domain of Bcr/Abl is required for efficient induction of chronic myeloid leukemia-like disease in mice but not for lymphoid leukemogenesis or activation of phosphatidylinositol 3-kinase. Blood 2001;97:4-13. [Crossref] [PubMed]
- Parikh C, Subrahmanyam R, Ren R. Oncogenic NRAS rapidly and efficiently induces CMML- and AML-like diseases in mice. Blood 2006;108:2349-57. [Crossref] [PubMed]
- Kong Y, Chang YJ, Liu YR, et al. CD34(+)CD38(-)CD58(-) cells are leukemia-propagating cells in Philadelphia chromosome-positive acute lymphoblastic leukemia. Leukemia 2014;28:2398-401. [Crossref] [PubMed]
- Herrero A, Pinto A, Colón-Bolea P, et al. Small Molecule Inhibition of ERK Dimerization Prevents Tumorigenesis by RAS-ERK Pathway Oncogenes. Cancer Cell 2015;28:170-82. [Crossref] [PubMed]
- Zhou F, Li X, Wang W, et al. Tracing haematopoietic stem cell formation at single-cell resolution. Nature 2016;533:487-92. [Crossref] [PubMed]
- Brentjens RJ, Davila ML, Riviere I, et al. CD19-targeted T cells rapidly induce molecular remissions in adults with chemotherapy-refractory acute lymphoblastic leukemia. Sci Transl Med 2013;5:177ra38. [Crossref] [PubMed]
- Kochenderfer JN, Dudley ME, Kassim SH, et al. Chemotherapy-refractory diffuse large B-cell lymphoma and indolent B-cell malignancies can be effectively treated with autologous T cells expressing an anti-CD19 chimeric antigen receptor. J Clin Oncol 2015;33:540-9. [Crossref] [PubMed]
- Porter DL, Levine BL, Kalos M, et al. Chimeric antigen receptor-modified T cells in chronic lymphoid leukemia. N Engl J Med 2011;365:725-33. [Crossref] [PubMed]
- Amrolia PJ, Pule M. Chimeric antigen receptor T cells for ALL. Lancet 2015;385:488-90. [Crossref] [PubMed]
- Lee DW, Barrett DM, Mackall C, et al. The future is now: chimeric antigen receptors as new targeted therapies for childhood cancer. Clin Cancer Res 2012;18:2780-90. [Crossref] [PubMed]
- Kershaw MH, Westwood JA, Darcy PK. Gene-engineered T cells for cancer therapy. Nat Rev Cancer 2013;13:525-41. [Crossref] [PubMed]
- Maude SL, Teachey DT, Porter DL, et al. CD19-targeted chimeric antigen receptor T-cell therapy for acute lymphoblastic leukemia. Blood 2015;125:4017-23. [Crossref] [PubMed]
Cite this article as: Li D, Zhao X, Zhang R, Jiao B, Liu P, Ren R. BCR/ABL can promote CD19+ cell growth but not render them long-term stemness. Stem Cell Investig 2016;3:85.