Mini and customized low-cost bioreactors for optimized high-throughput generation of tissue organoids
Organoids are three-dimensional (3D) tissue-like constructs that differentiate from stem cell-derived, self-assembled embryoid bodies to closely resemble the complex environment, architecture, and function of the native tissue. The cellular microenvironment produced by organoids vastly outclasses the capacity of a standard 2D cell culture plate to simulate the intricacies of living biological systems. With striking similarity to complex living tissues in terms of organization, orientation, and behavior of the constituent heterogeneous cell types, stem cell-derived organoids represent the cutting-edge technology in in vitro biology. A flurry of recent publications detailing ever-improving methodologies and applications for organoids, including those from patient-derived induced pluripotent stem cells (iPSCs), herald an exciting new frontier of human disease research and personalized medicine.
In the context of this editorial, a bioreactor is defined as a dynamic 3D cell culture platform for a variety of distinct biomedical applications, such as: expansion of diverse cell types including PSCs, generation of 3D organoids from PSCs, and tissue-specific differentiation of stem cell-derived organoids (1). Bioreactors appear to offer a better venue for maintenance and differentiation of organoids because of the flow of media around the cells. This dynamic process provides an improved exchange of media at the surface of the organoid, ensuring higher mass-transfer and more physiologically relevant gradients of nutrients and waste products between the surface and the core (2,3). This gradient enables correct orientation and lamination of different cell phenotypes during differentiation by more closely mimicking the natural developmental environment (4,5). Furthermore, the shear imparted by flowing media can induce mechanotransducive responses from cells, leading to better structural and transcriptional outcomes (6). The complex environments provided by bioreactors can permit optimized conditions for the formation of embryoid bodies and their subsequent differentiation into mature tissues (7). During differentiation, individual tissue types might have distinctive requirements for growth factors, nutrient exchange, shear forces, volumes, and proximity to other cells. To address such an extensive diversity, a multitude of bioreactor classes with unique functionalities have been designed for organoid generation and maintenance (Figure 1). Each form of bioreactor possesses discrete advantages and disadvantages, as illustrated in Table 1.
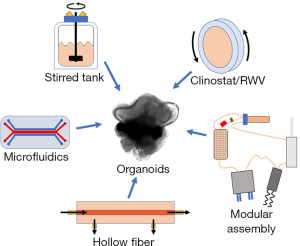
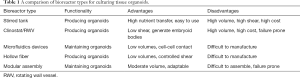
Full table
A relatively popular bioreactor system for organoid culture is the rotating wall vessel (RWV) or clinostat bioreactor. The RWV bioreactor, initially designed by NASA to simulate certain aspects of microgravity, functions by optimally suspending organoids in a low-turbulence environment that rotates horizontally with the fluid-filled bioreactor as a “solid-body” (8). Minor perturbations to the path of travel provide high mass-transfer under extremely low-shear conditions. A continuously-perfused RWV bioreactor was utilized to improve the rate of proliferation, cell-specific differentiation, and overall organization of murine retinal organoids (9). This study demonstrated that organoids in the RWV reached the same level of maturation in 25 days (9) compared with 32 days in a static culture (10). Additionally, organoids cultured in the RWV bioreactor had better differentiation of ganglion cells and S-cone photoreceptors, closely recapitulating a native retina. Further validating the significance of bioreactors, another report showed how culture in a stirred-tank bioreactor improved lamination and photoreceptor yield in human retinal organoids (11). The stirred-tank bioreactor employs a spinning propeller to suspend organoids in vigorously mixed media, exchanging higher shear for better nutrient exchange versus the RWV. In a live retina, phototransduction, the process of light being converted into electrical signals, occurs in the outer segment. A significantly higher proportion of photoreceptor cells in organoids produced in the stirred-tank bioreactor seemed to possess outer segments compared with those grown in static culture.
The improved organization and behavior of cells in bioreactor-cultured retinal organoids was evident in several recent studies involving an array of tissue types (12,13). However, many modern bioreactors are quite large; their prolonged used is frequently cost-prohibitive, potentially consuming liters of expensive growth factor-containing medium during a single experiment. A traditional perfusion RWV and a stirred-tank bioreactor may each hold up to 100 mL. The entirety of the media may need to be changed between each stage of differentiation, rapidly escalating the cost. Additionally, bioreactors are often not purpose-built for specific organ-type applications and are frequently skill- and experience- limiting. Until recently, researchers had endeavored to optimize bioreactors for 3D tissue culture (14,15) but were limited due to availability of differentiation protocols and advanced fabrication techniques. In the new era of 3D printing and mass customization, new generations of bioreactors are being developed that offer optimized organoid culture at reduced cost and improved reproducibility.
A prime example of this trend was implemented in a novel 3D-printed bioreactor assembly that is capable of converting each well of a standard 12-well tissue culture plate into a miniaturized, stirred-flask bioreactor (16). The abilities of the new bioreactor, named SpinΩ, were exemplified through the generation of human iPSC-derived, brain-region specific organoids. Purpose-built for low volumes, the SpinΩ possesses novel 3-fin vanes capable of continuously suspending organoids in just 3 mL of media each. These organoids exhibited a similar distribution of cell types and structures as the traditional protocols but with improved consistency. This or similar low-volume, multi-well setups provide a glimpse of the wide-range of options possible through customization and should be ideal for high-throughput applications of organoid research.
Notably, the customization is not limited to stirred-tank bioreactors. Another recent report described a modified microfluidics chip, which affixed neural organoids adjacent to media-perfusing channels for high mass transfer (17). This device enables the growth of organoids under extremely controlled, very low media volume conditions and confers similar benefits to differentiation and gene expression as those described above. As another example for innovative miniaturized bioreactors, a calcium alginate hollow fiber was used to produce very large human brain organoids, with preferable structural and phenotypic results (18).
The designs of recently-published bioreactors for organoid production vary widely in their form and function, reflecting divergent tissue-specific requirements for organoid generation and for broad and ever-expanding applications. For full capacity of customized bioreactors to be realized, stem cell biologists and engineers will need to continue developing an array of new systems. The next generation of cell culture bioreactors will require more rigorous consistency and lower cost, in addition to introducing novel designs relevant to developmental biology. Such alterations may include manipulations of oxygen concentrations, asymmetric growth factor treatments, and systematic incorporation of extracellular matrix proteins or mature cell types such as endothelial cells. With the intensity of interest in organoid research, cost reduction and customization are inevitable to propel organoids to the same level of prominence, ubiquity, and reproducibility as basic 2D cell culture systems.
Acknowledgements
We thank Holly Chen for providing the retina organoid picture.
Funding: Our research is supported by Intramural Research program of the National Eye Institute (EY000450, EY000474, EY000546). PIL acknowledges support by the National Aeronautics and Space Agency (NASA), Grant # 80NSSC18K1480.
Footnote
Conflicts of Interest: The authors have no conflicts of interest to declare.
References
- Selden C, Fuller B. Role of Bioreactor Technology in Tissue Engineering for Clinical Use and Therapeutic Target Design. Bioengineering (Basel) 2018.5. [PubMed]
- Sant S, Johnston PA. The production of 3D tumor spheroids for cancer drug discovery. Drug Discov Today Technol 2017;23:27-36. [Crossref] [PubMed]
- Van Winkle AP, Gates ID, Kallos MS. Mass transfer limitations in embryoid bodies during human embryonic stem cell differentiation. Cells Tissues Organs 2012;196:34-47. [Crossref] [PubMed]
- McMurtrey RJ. Analytic Models of Oxygen and Nutrient Diffusion, Metabolism Dynamics, and Architecture Optimization in Three-Dimensional Tissue Constructs with Applications and Insights in Cerebral Organoids. Tissue Eng Part C Methods 2016;22:221-49. [Crossref] [PubMed]
- Yin X, Mead BE, Safaee H, et al. Engineering Stem Cell Organoids. Cell Stem Cell 2016;18:25-38. [Crossref] [PubMed]
- Wieck MM, Schlieve CR, Thornton ME, et al. Prolonged Absence of Mechanoluminal Stimulation in Human Intestine Alters the Transcriptome and Intestinal Stem Cell Niche. Cell Mol Gastroenterol Hepatol 2017;3:367-88.e1. [Crossref] [PubMed]
- Gerecht-Nir S, Cohen S, Itskovitz-Eldor J. Bioreactor cultivation enhances the efficiency of human embryoid body (hEB) formation and differentiation. Biotechnol Bioeng 2004;86:493-502. [Crossref] [PubMed]
- Hammond T, Hammond J. Optimized suspension culture: the rotating-wall vessel. Am J Physiol Renal Physiol 2001.281. [PubMed]
- DiStefano T, Chen HY, Panebianco C, et al. Accelerated and Improved Differentiation of Retinal Organoids from Pluripotent Stem Cells in Rotating-Wall Vessel Bioreactors. Stem Cell Reports 2018;10:300-13. [Crossref] [PubMed]
- Chen HY, Kaya KD, Dong L, et al. Three-dimensional retinal organoids from mouse pluripotent stem cells mimic in vivo development with enhanced stratification and rod photoreceptor differentiation. Molecular Vision 2016;22:1077-94. [PubMed]
- Ovando-Roche P, West EL, Branch MJ, et al. Use of bioreactors for culturing human retinal organoids improves photoreceptor yields. Stem Cell Res Ther 2018;9:156. [Crossref] [PubMed]
- Fatehullah A, Tan SH, Barker N. Organoids as an in vitro model of human development and disease. Nat Cell Biol 2016;18:246-54. [Crossref] [PubMed]
- Lancaster MA, Knoblich JA. Organogenesis in a dish: modeling development and disease using organoid technologies. Science 2014;345. [Crossref] [PubMed]
- Cimetta E, Figallo E, Cannizzaro C, et al. Micro-bioreactor arrays for controlling cellular environments: design principles for human embryonic stem cell applications. Methods 2009;47:81-9. [Crossref] [PubMed]
- Freed LE, Vunjak-Novakovic GV, Drinker PA, et al. Bioreactor Based on Suspended Particles of Immobilized Enzyme. Annals of Biomedical Engineering 1993;21:57-65. [Crossref] [PubMed]
- Qian X, Jacob F, Song MM, et al. Generation of human brain region–specific organoids using a miniaturized spinning bioreactor. Nature Protocols 2018;13:565. [Crossref] [PubMed]
- Wang Y, Wang L, Guo Y, et al. Engineering stem cell-derived 3D brain organoids in a perfusable organ-on-a-chip system. RSC Advances 2018;8:1677-85. [Crossref]
- Zhu Y, Wang L, Yin F, et al. A hollow fiber system for simple generation of human brain organoids. Integr Biol (Camb) 2017;9:774-81. [Crossref] [PubMed]
Cite this article as: Phelan MA, Lelkes PI, Swaroop A. Mini and customized low-cost bioreactors for optimized high-throughput generation of tissue organoids. Stem Cell Investig 2018;5:33.