Adverse events, side effects and complications in mesenchymal stromal cell-based therapies
Introduction
The past several decades have seen the rapid development of cell-based regenerative medicine (1,2). Cell therapy has shown increasing advances with numerous cell-products based on autologous peripheral blood lymphocytes, allogeneic or autologous mesenchymal stromal cells (MSCs), hematopoietic cells, fibroblasts, chondrocytes, etc. (3,4). MSCs were recognised as easy derivable and the most applicable choice among the other cell-sources for a wide range of pathologies (5-10). These cells are commonly obtained from the bone marrow (11), adipose tissue (12), umbilical cord (13), dental pulp (14), gingiva (15), perinatal tissues (16), etc. Cell profile of low differentiated MSCs is generally characterized as positive for CD73, CD105 and CD90 cell surface markers and negative for hematopoietic markers CD19, CD20, CD34 and CD45 (17). MSCs secrete multiple growth factors and cytokines that also determine their regenerative effect. In particular, being derived from human umbilical cord, MSCs release keratinocyte growth factor (KGF) (18), hepatocyte growth factor (HGF) (19), transforming growth factor-β (TGF-β) (20) and exosomes, which suppress inflammation and lead to improved vascularization and epithelialization in affected tissues (21).
The proliferation of MSCs-related studies created a ‘cure-all’ paradigm with a trend to apply MSCs for the treatment of various diseases: leukemia, anemia, autoimmune, degenerative and cardiovascular diseases, and malignant tumors (22). Recently, MSC-based cell therapy has even been used to treat coronavirus disease 2019 (COVID-19) (23,24). However, novel clinical trials dramatically increased the reports on adverse events and side effects of MSCs-based therapy. In some cases, the effectiveness of cell therapy appears to be overestimated, which potentially may lead to tragic tolls in patient outcomes (25,26).
We aimed to overview and systemate all the adverse events and side effects reported after clinical application of MSCs. Some of them occurred due to the nature of this kind of cells, and others may be related to MSCs-cell culture conditions (Figure 1). Thus, we are raising clinical and scientific awareness on reported adverse events and side effects.
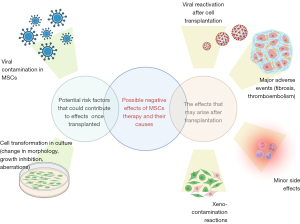
Cell transformation in culture (change in morphology, growth inhibition, aberrations)
Cells are capable of transforming in culture due to modification of their regulatory activity by growth factors, cell medium, adhesion, and intercellular connections. Culture-expanded MSCs exhibit notable differences in terms of cell morphology, physiology, and function, which decisively contribute to the heterogeneity of MSC’s (27). The heterogeneity of MSCs during in vitro expansion led to morphological alterations, modifications in the gene and protein expression profile, discrepancies in the differentiation potential, and physiological changes in cells (28,29) The majority of cell cultures are characterized by heterogeneity in subpopulations due to the influence of multiple factors arising during protocol reproduction. In particular, the cell-phenotype of MSCs are influenced by confluence density at the end of each passage (30). The density of cell confluence in bone marrow MSC culture affects the expression of more than two thousand genes, leading to changes in cell profile and cytokine synthesis (31). Thus, cell products and experimental models reproducible under the same conditions may have unpredictable affinity. However, scientists generally still use a qualitative assessment of the monolayer density when checking one or several fields under the microscope. These factors may change cell profile in products prepared for clinical application, causing adverse events.
Cell proliferation in vitro may be associated with replicative stress. Kim et al. showed that in MSCs, the majority of single-nucleotide variations (C > A transversions) occur in late passages (P7-P9) (32).
Importantly, the number of passages does not equal the cell divisions. Usually passing means that cellular monolayer grown on a dish bottom plated on the same dishes as 1:4. This cell concentration completely covers the bottom of the dish within one week.
Røsland et al. reported a spontaneous malignant transformation of approximately 46% of human MSC after four weeks in culture (33). A similar effect was observed for monkey MSCs after long-term cultivation (34). Transformed cells showed changes in growth and morphology similar to those of tumor cells. Furthermore, subcutaneous administration of transformed cells into NOD/SCID mice led to tumor formation.
Some studies noted that long-term cell culture leads to accumulation of chromosomal abnormalities. Froelich et al. reported that chromosomal abnormalities in adipose-derived MSCs increased significantly starting from passage 5 (35). In general, chromosomal aberrations occur in human MSCs in 4% of cases (primary monosomies) compared to 9% (primary trisomies) in pluripotent stem cells and neuronal stem cells (36). Research by Omel’chenko et al. showed that human MSCs with a perivascular immunophenotype during cell culture expressed the HSPB6, PLAC9, FEZ1, DTWD1, APH1A, and ATP5L genes associated with cell transformation (37).
However, human MSCs obtained from chorionic villi and amniotic fluid or umbilical cord (hUC-MSC) showed no signs of malignant transformation or chromosomal aberrations after in vitro culture up to the 15th passage (38,39). hUC-MSC cells in different passages had similar morphology, biomarker expression, and cytokine secretion (40).
In vitro cultivation of MSCs does not always cause chromosomal abnormalities, however, they have been reported in <10 passage cells conditioning the need for examination of MSCs before clinical use in order to avoid undesirable consequences.
Viral and mycoplasma containment in MSCs
When using allogeneic MSCs, donors are routinely tested for the presence of viruses such as human immunodeficiency virus (HIV), however, transplanted MSCs can contain genetic material of other types of viruses (41). According to Sundin et al. MSCs derived from various tissues may contain persistent viruses (42). The authors found that the MSCs derived from healthy donors contained viral DNA of parvovirus B19. Viral DNA has also been found in human bone marrow (43). The most common were B19V and torque teno virus (TTV): 62.9%, Epstein-Barr virus (EBV): 14.8%, Merkel cell polyomavirus (MCPyV): 11.1%, and human herpesvirus 7 (HHV-7): 7.4%.
MSCs may be infected with various viruses, among which respiratory syncytial virus (RSV), avian influenza A H5N1 (44,45). The presence of viruses in MSCs may be caused not only by viral infection of a cell donor. Viral contamination of different types of cells may also occur during cell processing and culture (46). For this reason, when working with MSCs, it is necessary to strictly follow the established requirements in order to avoid viral contamination.
Mycoplasma contamination can also be a serious threat for cultured cells. Interruptions of laminar flow, incomplete sterilization cycles, inappropriate lab clothes (dirty lab coats, reuse of same gloves, etc.) and enhanced doses of antibiotics in culture can lead to mycoplasma contamination (47). Cryopreservation in Dewar tanks containing viral or mycoplasma contaminated cell-probes may cross-contaminate other cells even when infected and ‘healthy’ cells are stored in different cryovials (48,49).
Xeno-contamination reactions caused by components of culture medium
Xeno-contamination reactions are rare and commonly associated with the presence of supportive xenogenic components in cell products. Commonly cryopreservation (50,51) and expanding media containing fetal bovine serum (FBS), human serum albumin, or patient’s autologous plasma (52-54). Today, FBS is excluded from the majority of clinical protocols due to the risk of disease transmission and xenogeneic immune reactions and replaced by alternative protein sources (55,56). Currently, autologous blood plasma could be considered a ‘gold standard’ for cell cryopreservation in clinical practice, which has been well studied since the 1990s (57,58). Human cord blood plasma has also recently been described among the novel alternative FBS compounds for MSC culture (59-62).
Major adverse events after cell injection: thromboembolism and fibrosis
The issue of numerous reported adverse events has to receive considerable critical attention. Some of them could be interpreted as the result of disease progression despite the provided cell-therapy with insufficient therapeutic effect. Thus, loss of vision was a negative reaction to adipose-derived MSC therapy in three patients with age-related macular degeneration (63). In another study, MSCs therapy alone does not lead to the full-thickness restoration of ulcerated skin after topical administration (64). The effectiveness of adipose- and bone marrow-derived MSC in the treatment of lateral amyotrophic sclerosis is also controversial. For example, a clinical trial conducted by Syková et al. revealed a slow down in disease progression only in some patients within 6 months after bone marrow-derived MSC application (65). Another clinical trial did not show any positive effects after adipose-derived MSC therapy (66). This raises the point of actual clinical significance of MSCs for tissue repair. Furthermore, MSCs caused side effects in 12% of cases after COVID-19 treatment in a small cohort of patients. The safety and effectiveness of COVID-19 treatment with MSCs therapy were criticized in the sense of known blood clotting effects triggered by MSCs. In particular MSCs could promote tromboembolism via release of procoagulant tissue factor (10). In general, reported adverse events also included liver dysfunction, heart failure, and allergic rash, which are typical complications of severe pneumonia (23,67).
Wu et al. reported two cases of inflammatory associated thromboembolism in kidney transplant patients and chronic kidney disease following after infusion of hUC-MSC (68). Importantly, the overdosing of cell therapies is more likely to cause glomerular and tubular damage in the kidneys (69). MSCs-therapy may also cause pulmonary embolism (70). Importantly, the risks of thromboembolization, as well as effectiveness of cell therapy, were shown to be determined by the recipient anamnesis and phenotyping features (71).
Večerić-Haler et al. described a clinical case of a complication that mimics capillary leak syndrome with ultimate kidney graft failure after autologous MSCs transplantation in the patient with a history of acute lymphoblastic leukemia (72).
However, we have to distinguish these cases from the following major adverse events, which appeared due to weakly controllable differentiation of MSCs. Recently, interstitial tissue fibrosis and tubular atrophy have been observed in a patient with chronic kidney disease following infusion of autologous adipose-derived MSCs (73). Importantly, the capability of MSCs of differentiation into myofibroblasts with the development of fibrous tissue was already well-demonstrated in previous experimental studies (74).
In the multicenter study, 2,372 patients with degenerative joint disease treated with autologous MSCs injections were enrolled (75). The majority of adverse events were just post-procedure pain or complications of the treated disease. However, neoplasms, neurologic, and vascular signs were among the serious adverse events. The authors reported about 7 cases of neoplasms that represent 0.3% of the study population, with an incidence of 0.14/100 PY. However, the authors disclaimed associations between the neoplasms and cell therapy. Serious neurologic and vascular events were 6 and 5 cases, respectively, representing 0.25% and 0.21% of the total population.
The well-known suppression of immune responses determined by MSCs explains the success of MSC therapy for graft-versus-host reactions and other various autoimmune disorders (76). Subsequently, systemic administration of MSCs caused major adverse events due to their immunosuppressive properties. In particular, MSCs therapy increases the risk of pneumonia-related death after allogeneic hematopoietic stem cell (HSC) transplantation (77). Interestingly, stem cell transplantation is also related to alterations in different lymphocyte populations (CD4+ T-helper cells, CD8+ T-cells, CD19+ and CD20+ B-lymphocytes). The CD4/CD8 ratio as well as CD19+ and CD20+ cell populations decreased in patients responded to stem cell therapy (78).
Minor side effects after cell injection: fever and local pain
The majority of clinical studies report that the use of MSCs is safe and feasible, with only minor side effects. The most common example is fever that occurred in 22% of patients following hUC-MSC infusion for COVID-19 treatment (79), in 9.8% of patients after the hUC-MSCs therapy for treatment of Crohn’s disease (80), in 85% of patients with progressive multiple sclerosis after treatment of autologous BM-MSCs (81). The use of allogeneic MSCs for the treatment of liver failure caused fever in 19.2% of patients during 5–24 weeks of follow-up. One of the causes of fever was supposed to be a reaction to the residual phosphate-buffered saline (PBS) buffer (82). Meta-analysis of the prospective clinical trials of intravascular injections of MSCs identified a significant association between MSCs and transient fever (83). Intra-articular injection of MSC mild effusion and increased local pain in patients within 48–72 hours (84).
Effects of MSC’s cell therapy on neoplasms
Oncological anamnesis remains a well-known exclusion criterion for clinical studies, explained by the controversial impact of MSCs on the tumor growth and behavior. Nevertheless, some publications suggested that the MSCs administration had no effect on the tumor, or even inhibited its growth in vivo (85,86). However, the study by Ning et al. reported a statistically significant increase in relapse rates (60% vs. 20%, P=0.02) and a decrease in 3-year relapse-free survival (30% vs. 66.7%, P=0.035) in patients co-transplanted with HSCs and culture-expanded MSCs versus patients transplanted with HSCs only. The authors pointed out the need for additional large-scale randomized clinical trials in order to evaluate the potential benefits and hazards of MSC-cotransplantation in malignant hematopoietic diseases (87). In addition, some animal and in-vitro studies showed that MSCs administration may promote growth of different tumors through a variety of mechanisms, including expression of proangiogenic factors and immunosuppression (88,89). We consider it crucial to perform a comprehensive patient examination in order to find possible malignancy and exclude patients before initiation of a cell-based therapy. However, it is vital to understand that there are more than 100 types of cancer-related diseases (90), and that tumor diagnostics remains a complicated task and not always results in the identification of neoplasms at early-stages, imposing certain risks and limiting cell-based therapy. Safety concerns gave us reason to suggest that currently, cell-based therapy should be administered with great precaution in cases where the possible benefit outweighs the risk (Table 1).
Table 1
Adverse events and side effects | Causes | Implications | Refs |
---|---|---|---|
Cell transformation in culture (change in morphology, growth inhibition, aberrations) | Long-term culturing (10+ passages) Mutagenic components of cell media Cells differentiation in culture with specific antigen presenting |
Systemic immunogenic responses on injected cells Local inflammation and prolonged immunological reactions |
(27-40) |
Viral containment in MSCs | Cryobanking with contaminated cultures Violations of biosafety protocols during cell processing and culture |
Cellular dysfunction Viral infection Infection complications after injection |
(41-49) |
Xeno-contamination reactions | Exogenous growth factors in media Using of cell media contained animal serum |
Acute inflammation | (50-62) |
Major adverse events after cell injection: thromboembolism and fibrosis | Injection of cell aggregates Personal phenotyping properties Personal sensitivity |
Pulmonary and renal thromboembolism Cardiac and liver fibrosis |
(10,23,63-78) |
Minor side effects after cell injection | The injection contains remnants of the PBS buffer; Intra-articular injection | Fever; local pain | (79-84) |
Effects on tumors and neoplasms | Expression of proangiogenic factors; immunosuppression | The formation of malignant neoplasms | (85-90) |
MSC, mesenchymal stromal cell; PBS, phosphate-buffered saline.
Discussion
One of the major tasks is to ensure the effectiveness of treatment and patients safety under cell therapy. Currently limited success of MSCs-therapy could be a subsequence of transformations during cell-culture or the result of major adverse events appearing due to uncontrollable differentiation. Notably, MSCs exhibit advanced pro chondrogenic, osteogenic, and adipogenic differentiation potential. Manifestations of their mis-differentiation were evaluated in multiple animal studies. Direct transplantation of unselected bone marrow cells into the acutely infarcted myocardium was shown to induce significant intramyocardial calcification (91). Liao et al. indicated the calcification of the injured abdominal aorta after BM-MSC administration in the experimental rat model (92). Several studies reported that BM-MSC precultured under hypoxic conditions demonstrated superior vascularisation effects via miR-16 (93). Those cells also had enhanced migratory and self-renewal or clonogenic capacities (94,95). However, besides the regenerative advantages, scientists have shown increased activity in the mTOR signaling pathway leading to autophagy. Furthermore, hypoxic pre-cultured MSCs had pro-senescent cell profile and up-regulated activity of superoxide dismutase consequenced in inhibited apoptosis (96,97). Thus, routine hypoxic cell culture for therapeutic administration requires further in-depth research of modulated gene expression and metabolic changes.
The majority of clinical studies with MSCs have included a limited number of patients, which may affect the assessment of statistical significance (Figure 2). The major adverse events were reported as presumably associated with systematic intravascular administration of low differentiated cells (i.e., MSCs) (98-100). Essential part of performed studies administered MSCs in combination with biomaterials and biomolecules, that masked therapeutic effect of cells. An additional factor may have been the lack of predictive methods and quantitative approaches in the planning of cell therapies (27,101,102).
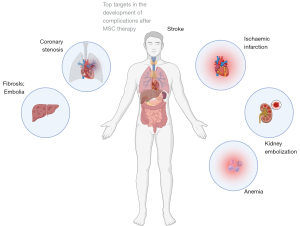
Therapeutic effect and frequency of complications after multipotent cell therapy remain questionable (103-105). The therapeutic effects are generally based on anti-inflammatory effect caused by prolonged paracrine activity of injected cells (106). Thus, special attention should be paid to the age of the donor. MSCs donated by elderly patients have altered immunomodulatory profile. Adipose-derived MSCs obtained from elderly donors with atherosclerosis were characterized by a higher level of proinflammatory cytokines IL-6 and IL-8 (107).
Multiple complications can be explained by the mesenchymal phenotype causing their migration and adhesion. Unlike multipotent cells, the use of highly differentiated somatic cells remains harmless. Hence, we conceive that highly differentiated cells could be a feature ‘gold standard’ in regenerative medicine. The reported major adverse events were generally caused by inappropriate understanding of risk factors associated with stem cell therapy. In accordance with observed clinical reports we designed a short checklist, recommended for acceptance in all cases of MSCs transplantation (Table 2).
Table 2
Before every MSCs transplantation check whether: |
The donor’s anamnesis contains no information about any infectious diseases |
MSCs are under 9 passages |
PCR testing for mycoplasma and herpesviruses were performed |
Cryobanking dewar contain no untested (i.e., mycoplasma and HHV-6) or unknown cell probes |
Cell quantity and concentration are adjusted and do not exceed the necessary therapeutic limits |
Way of systemic delivery have been chosen in accordance to expected cell homing |
Antihistamine and anti-inflammation therapy is prepared and not contraindicated |
MSC, mesenchymal stromal cell; PCR, polymerase chain reaction; HHV-6, human herpesvirus 6.
Another effective alternative could be non-cultured cells, cryopreserved immediately after harvesting. This type is already well-known as minimally manipulated cells. Recent trends in legal regulations all over the world already paved the way for wide spreading of minimally manipulated cells for clinical application as a safe and effective alternative to pre-cultured cells with an unpredictable risk of phenotypic changes (108). However, regenerative products based on minimally manipulated MSCs generally contain mesenchymal stromal fraction with a minor presence of CD90-positive low differentiated cells (109).
MSCs-derived extracellular vesicles also appeared as a novel therapeutic alternative to cultured cells (110,111). Exosomes could be collected either from normal or from hypoxic cell culture (112). The advantages and limitations of promising cell-free regenerative medicine are expected to be in a focus field of upcoming publications.
Conclusions
Our short review set out with the aim of assessing the safety of stem cell therapy with low differentiated cells. We observed numerous adverse events associated with uncontrollable cell differentiation and transformation. We found that safety and feasibility of stem cell therapy are still weakly estimated in cases with low differentiated and multipotent cells. Currently, the privilege in clinical trials should be addressed to minimally manipulated and high-differentiated cells. Despite many positive reports, MSCs therapy remains a risky therapy with delayed adverse effects. Their manifestation generally depends on the manufacturing quality and patient individual phenotype. Possible complications should be considered for the planning and administration of clinical trials with obligatory inclusion in the patient’ informed consents.
Acknowledgments
The figures were generated using biorender.com.
Funding: None.
Footnote
Conflicts of Interest: All authors have completed the ICMJE uniform disclosure form (available at https://sci.amegroups.com/article/view/10.21037/sci-2022-025/coif). The authors have no conflicts of interest to declare.
Ethical Statement: The authors are accountable for all aspects of the work in ensuring that questions related to the accuracy or integrity of any part of the work are appropriately investigated and resolved.
Open Access Statement: This is an Open Access article distributed in accordance with the Creative Commons Attribution-NonCommercial-NoDerivs 4.0 International License (CC BY-NC-ND 4.0), which permits the non-commercial replication and distribution of the article with the strict proviso that no changes or edits are made and the original work is properly cited (including links to both the formal publication through the relevant DOI and the license). See: https://creativecommons.org/licenses/by-nc-nd/4.0/.
References
- Yamada S, Behfar A, Terzic A. Regenerative medicine clinical readiness. Regen Med 2021;16:309-22. [Crossref] [PubMed]
- Rajabzadeh N, Fathi E, Farahzadi R. Stem cell-based regenerative medicine. Stem Cell Investig 2019;6:19. [Crossref] [PubMed]
- Morgan RA, Yang JC, Kitano M, et al. Case report of a serious adverse event following the administration of T cells transduced with a chimeric antigen receptor recognizing ERBB2. Mol Ther 2010;18:843-51. [Crossref] [PubMed]
- van den Berg JH, Gomez-Eerland R, van de Wiel B, et al. Case Report of a Fatal Serious Adverse Event Upon Administration of T Cells Transduced With a MART-1-specific T-cell Receptor. Mol Ther 2015;23:1541-50. [Crossref] [PubMed]
- Krasilnikova OA, Baranovskii DS, Lyundup AV, et al. Stem and Somatic Cell Monotherapy for the Treatment of Diabetic Foot Ulcers: Review of Clinical Studies and Mechanisms of Action. Stem Cell Rev Rep 2022;18:1974-85. [Crossref] [PubMed]
- Baranovskii DS, Akhmedov BG, Demchenko AG, et al. Minimally Manipulated Bone Marrow-Derived Cells Can Be Used for Tissue Engineering In Situ and Simultaneous Formation of Personalized Tissue Models. Bull Exp Biol Med 2022;173:139-45. [Crossref] [PubMed]
- Ringdén O, Moll G, Gustafsson B, et al. Mesenchymal Stromal Cells for Enhancing Hematopoietic Engraftment and Treatment of Graft-Versus-Host Disease, Hemorrhages and Acute Respiratory Distress Syndrome. Front Immunol 2022;13:839844. [Crossref] [PubMed]
- Moll G, Ankrum JA, Kamhieh-Milz J, et al. Intravascular Mesenchymal Stromal/Stem Cell Therapy Product Diversification: Time for New Clinical Guidelines. Trends Mol Med 2019;25:149-63. [Crossref] [PubMed]
- Moll G, Ankrum JA, Olson SD, et al. Improved MSC Minimal Criteria to Maximize Patient Safety: A Call to Embrace Tissue Factor and Hemocompatibility Assessment of MSC Products. Stem Cells Transl Med 2022;11:2-13. [Crossref] [PubMed]
- Moll G, Drzeniek N, Kamhieh-Milz J, et al. MSC Therapies for COVID-19: Importance of Patient Coagulopathy, Thromboprophylaxis, Cell Product Quality and Mode of Delivery for Treatment Safety and Efficacy. Front Immunol 2020;11:1091. [Crossref] [PubMed]
- Jeyaraman M, Bingi SK, Muthu S, et al. Impact of the Process Variables on the Yield of Mesenchymal Stromal Cells from Bone Marrow Aspirate Concentrate. Bioengineering (Basel) 2022;9:57. [Crossref] [PubMed]
- Cheng NC, Estes BT, Awad HA, et al. Chondrogenic differentiation of adipose-derived adult stem cells by a porous scaffold derived from native articular cartilage extracellular matrix. Tissue Eng Part A 2009;15:231-41. [Crossref] [PubMed]
- Hua J, Gong J, Meng H, et al. Comparison of different methods for the isolation of mesenchymal stem cells from umbilical cord matrix: proliferation and multilineage differentiation as compared to mesenchymal stem cells from umbilical cord blood and bone marrow. Cell Biol Int 2013; Epub ahead of print. [Crossref] [PubMed]
- Rajendran R, Gopal S, Masood H, et al. Regenerative potential of dental pulp mesenchymal stem cells harvested from high caries patient's teeth. J Stem Cells 2013;8:25-41. [PubMed]
- Kim D, Lee AE, Xu Q, et al. Gingiva-Derived Mesenchymal Stem Cells: Potential Application in Tissue Engineering and Regenerative Medicine - A Comprehensive Review. Front Immunol 2021;12:667221. [Crossref] [PubMed]
- Pollard RT, Salter I, Sanders RJ, et al. Southern Ocean deep-water carbon export enhanced by natural iron fertilization. Nature 2009;457:577-80. [Crossref] [PubMed]
- Billing AM, Ben Hamidane H, Dib SS, et al. Comprehensive transcriptomic and proteomic characterization of human mesenchymal stem cells reveals source specific cellular markers. Sci Rep 2016;6:21507. [Crossref] [PubMed]
- Bi ZM, Zhou QF, Geng Y, et al. Human umbilical cord mesenchymal stem cells ameliorate experimental cirrhosis through activation of keratinocyte growth factor by suppressing microRNA-199. Eur Rev Med Pharmacol Sci 2016;20:4905-12. [PubMed]
- Zhao C, Zhang L, Kong W, et al. Umbilical Cord-Derived Mesenchymal Stem Cells Inhibit Cadherin-11 Expression by Fibroblast-Like Synoviocytes in Rheumatoid Arthritis. J Immunol Res 2015;2015:137695. [Crossref] [PubMed]
- Dabrowski FA, Burdzinska A, Kulesza A, et al. Comparison of the paracrine activity of mesenchymal stem cells derived from human umbilical cord, amniotic membrane and adipose tissue. J Obstet Gynaecol Res 2017;43:1758-68. [Crossref] [PubMed]
- Kulakov A, Kogan E, Brailovskaya T, et al. Mesenchymal Stromal Cells Enhance Vascularization and Epithelialization within 7 Days after Gingival Augmentation with Collagen Matrices in Rabbits. Dent J (Basel) 2021;9:101. [Crossref] [PubMed]
- Capilla-González V, Herranz-Pérez V, Sarabia-Estrada R, et al. Editorial: Mesenchymal Stromal Cell Therapy for Regenerative Medicine. Front Cell Neurosci 2022;16:932281. [Crossref] [PubMed]
- Golchin A. Cell-Based Therapy for Severe COVID-19 Patients: Clinical Trials and Cost-Utility. Stem Cell Rev Rep 2021;17:56-62. [Crossref] [PubMed]
- Leng Z, Zhu R, Hou W, et al. Transplantation of ACE2- Mesenchymal Stem Cells Improves the Outcome of Patients with COVID-19 Pneumonia. Aging Dis 2020;11:216-28. [Crossref] [PubMed]
- McKelvey M, Saemundsson RJ. Developing innovation governance readiness in regenerative medicine: lessons learned from the Macchiarini crisis. Regen Med 2021;16:283-94. [Crossref] [PubMed]
- Julian K, Yuhasz N, Rai W, et al. Complications from "Stem Cell Tourism" in Neurology. Ann Neurol 2020;88:661-8. [Crossref] [PubMed]
- Olmedo-Moreno L, Aguilera Y, Baliña-Sánchez C, et al. Heterogeneity of In Vitro Expanded Mesenchymal Stromal Cells and Strategies to Improve Their Therapeutic Actions. Pharmaceutics 2022;14:1112. [Crossref] [PubMed]
- Moll G, Rasmusson-Duprez I, von Bahr L, et al. Are therapeutic human mesenchymal stromal cells compatible with human blood? Stem Cells 2012;30:1565-74. [Crossref] [PubMed]
- Moll G, Alm JJ, Davies LC, et al. Do cryopreserved mesenchymal stromal cells display impaired immunomodulatory and therapeutic properties? Stem Cells 2014;32:2430-42. [Crossref] [PubMed]
- Ho AD, Wagner W, Franke W. Heterogeneity of mesenchymal stromal cell preparations. Cytotherapy 2008;10:320-30. [Crossref] [PubMed]
- Ren J, Wang H, Tran K, et al. Human bone marrow stromal cell confluence: effects on cell characteristics and methods of assessment. Cytotherapy 2015;17:897-911. [Crossref] [PubMed]
- Kim M, Rhee JK, Choi H, et al. Passage-dependent accumulation of somatic mutations in mesenchymal stromal cells during in vitro culture revealed by whole genome sequencing. Sci Rep 2017;7:14508. [Crossref] [PubMed]
- Røsland GV, Svendsen A, Torsvik A, et al. Long-term cultures of bone marrow-derived human mesenchymal stem cells frequently undergo spontaneous malignant transformation. Cancer Res 2009;69:5331-9. [Crossref] [PubMed]
- Ren Z, Wang J, Zhu W, et al. Spontaneous transformation of adult mesenchymal stem cells from cynomolgus macaques in vitro. Exp Cell Res 2011;317:2950-7. [Crossref] [PubMed]
- Froelich K, Mickler J, Steusloff G, et al. Chromosomal aberrations and deoxyribonucleic acid single-strand breaks in adipose-derived stem cells during long-term expansion in vitro. Cytotherapy 2013;15:767-81. [Crossref] [PubMed]
- Ben-David U, Mayshar Y, Benvenisty N. Large-scale analysis reveals acquisition of lineage-specific chromosomal aberrations in human adult stem cells. Cell Stem Cell 2011;9:97-102. [Crossref] [PubMed]
- Omel'chenko DO, Rzhaninova AA, Gol'dshteĭn DV. Comparative transcriptome pairwise analysis of spontaneously transformed multipotent stromal cells from human adipose tissue. Genetika 2014;50:106-15. [PubMed]
- Poloni A, Maurizi G, Babini L, et al. Human mesenchymal stem cells from chorionic villi and amniotic fluid are not susceptible to transformation after extensive in vitro expansion. Cell Transplant 2011;20:643-54. [Crossref] [PubMed]
- Chen G, Yue A, Ruan Z, et al. Human umbilical cord-derived mesenchymal stem cells do not undergo malignant transformation during long-term culturing in serum-free medium. PLoS One 2014;9:e98565. [Crossref] [PubMed]
- Zhao Q, Zhang L, Wei Y, et al. Systematic comparison of hUC-MSCs at various passages reveals the variations of signatures and therapeutic effect on acute graft-versus-host disease. Stem Cell Res Ther 2019;10:354. [Crossref] [PubMed]
- Thanunchai M, Hongeng S, Thitithanyanont A. Mesenchymal Stromal Cells and Viral Infection. Stem Cells Int 2015;2015:860950. [Crossref] [PubMed]
- Sundin M, Lindblom A, Örvell C, et al. Persistence of human parvovirus B19 in multipotent mesenchymal stromal cells expressing the erythrocyte P antigen: implications for transplantation. Biol Blood Marrow Transplant 2008;14:1172-9. [Crossref] [PubMed]
- Toppinen M, Sajantila A, Pratas D, et al. The Human Bone Marrow Is Host to the DNAs of Several Viruses. Front Cell Infect Microbiol 2021;11:657245. [Crossref] [PubMed]
- Cheung MB, Sampayo-Escobar V, Green R, et al. Respiratory Syncytial Virus-Infected Mesenchymal Stem Cells Regulate Immunity via Interferon Beta and Indoleamine-2,3-Dioxygenase. PLoS One 2016;11:e0163709. [Crossref] [PubMed]
- Thanunchai M, Kanrai P, Wiboon-Ut S, et al. Tropism of avian influenza A (H5N1) virus to mesenchymal stem cells and CD34+ hematopoietic stem cells. PLoS One 2013;8:e81805. [Crossref] [PubMed]
- Barone PW, Wiebe ME, Leung JC, et al. Viral contamination in biologic manufacture and implications for emerging therapies. Nat Biotechnol 2020;38:563-72. [Crossref] [PubMed]
- Nikfarjam L, Farzaneh P. Prevention and detection of Mycoplasma contamination in cell culture. Cell J 2012;13:203-12. [PubMed]
- Joaquim DC, Borges ED, Viana IGR, et al. Risk of Contamination of Gametes and Embryos during Cryopreservation and Measures to Prevent Cross-Contamination. Biomed Res Int 2017;2017:1840417. [Crossref] [PubMed]
- Bielanski A. A review of the risk of contamination of semen and embryos during cryopreservation and measures to limit cross-contamination during banking to prevent disease transmission in ET practices. Theriogenology 2012;77:467-82. [Crossref] [PubMed]
- Cottle C, Porter AP, Lipat A, et al. Impact of Cryopreservation and Freeze-Thawing on Therapeutic Properties of Mesenchymal Stromal/Stem Cells and Other Common Cellular Therapeutics. Curr Stem Cell Rep 2022;8:72-92. [Crossref] [PubMed]
- Moll G, Geißler S, Catar R, et al. Cryopreserved or Fresh Mesenchymal Stromal Cells: Only a Matter of Taste or Key to Unleash the Full Clinical Potential of MSC Therapy? Adv Exp Med Biol 2016;951:77-98. [Crossref] [PubMed]
- Sarmiento M, Ramírez P, Parody R, et al. Advantages of non-cryopreserved autologous hematopoietic stem cell transplantation against a cryopreserved strategy. Bone Marrow Transplant 2018;53:960-6. [Crossref] [PubMed]
- Weinberg RS. Cryopreservation techniques and freezing solutions. In: Best Practices in Processing and Storage for Hematopoietic Cell Transplantation; 2018:63-72.
- Smagur A, Mitrus I, Ciomber A, et al. Comparison of the cryoprotective solutions based on human albumin vs. autologous plasma: its effect on cell recovery, clonogenic potential of peripheral blood hematopoietic progenitor cells and engraftment after autologous transplantation. Vox Sang 2015;108:417-24. [Crossref] [PubMed]
- Park S, Lee DR, Nam JS, et al. Fetal bovine serum-free cryopreservation methods for clinical banking of human adipose-derived stem cells. Cryobiology 2018;81:65-73. [Crossref] [PubMed]
- Fujisawa R, Mizuno M, Katano H, et al. Cryopreservation in 95% serum with 5% DMSO maintains colony formation and chondrogenic abilities in human synovial mesenchymal stem cells. BMC Musculoskelet Disord 2019;20:316. [Crossref] [PubMed]
- Galmés A, Besalduch J, Bargay J, et al. Cryopreservation of hematopoietic progenitor cells with 5-percent dimethyl sulfoxide at -80 degrees C without rate-controlled freezing. Transfusion 1996;36:794-7. [Crossref] [PubMed]
- Zinno F, Landi F, Scerpa MC, et al. Processing of hematopoietic stem cells from peripheral blood before cryopreservation: use of a closed automated system. Transfusion 2011;51:2656-63. [Crossref] [PubMed]
- Vlaski-Lafarge M, Chevaleyre J, Cohen J, et al. Discarded plasma obtained after cord blood volume reduction as an alternative for fetal calf serum in mesenchymal stromal cells cultures. Transfusion 2020;60:1910-7. [Crossref] [PubMed]
- Rallapalli S, Guhathakurta S, Bishi DK, et al. A critical appraisal of humanized alternatives to fetal bovine serum for clinical applications of umbilical cord derived mesenchymal stromal cells. Biotechnol Lett 2021;43:2067-83. [Crossref] [PubMed]
- Bui HTH, Nguyen LT, Than UTT. Influences of Xeno-Free Media on Mesenchymal Stem Cell Expansion for Clinical Application. Tissue Eng Regen Med 2021;18:15-23. [Crossref] [PubMed]
- Carnevale G, Pisciotta A, Riccio M, et al. Optimized Cryopreservation and Banking of Human Bone-Marrow Fragments and Stem Cells. Biopreserv Biobank 2016;14:138-48. [Crossref] [PubMed]
- Kuriyan AE, Albini TA, Townsend JH, et al. Vision Loss after Intravitreal Injection of Autologous "Stem Cells" for AMD. N Engl J Med 2017;376:1047-53. [Crossref] [PubMed]
- Maksimova NV, Michenko AV, Krasilnikova OA, et al. Mesenchymal stromal cell therapy alone does not lead to complete restoration of skin parameters in diabetic foot patients within a 3-year follow-up period. Bioimpacts 2022;12:51-5. [PubMed]
- Syková E, Rychmach P, Drahorádová I, et al. Transplantation of Mesenchymal Stromal Cells in Patients With Amyotrophic Lateral Sclerosis: Results of Phase I/IIa Clinical Trial. Cell Transplant 2017;26:647-58. [Crossref] [PubMed]
- Staff NP, Madigan NN, Morris J, et al. Safety of intrathecal autologous adipose-derived mesenchymal stromal cells in patients with ALS. Neurology 2016;87:2230-4. [Crossref] [PubMed]
- Chen X, Shan Y, Wen Y, et al. Mesenchymal stem cell therapy in severe COVID-19: A retrospective study of short-term treatment efficacy and side effects. J Infect 2020;81:647-79. [Crossref] [PubMed]
- Wu Z, Zhang S, Zhou L, et al. Thromboembolism Induced by Umbilical Cord Mesenchymal Stem Cell Infusion: A Report of Two Cases and Literature Review. Transplant Proc 2017;49:1656-8. [Crossref] [PubMed]
- Munk A, Duvald CS, Pedersen M, et al. Dosing Limitation for Intra-Renal Arterial Infusion of Mesenchymal Stromal Cells. Int J Mol Sci 2022;23:8268. [Crossref] [PubMed]
- Jung JW, Kwon M, Choi JC, et al. Familial occurrence of pulmonary embolism after intravenous, adipose tissue-derived stem cell therapy. Yonsei Med J 2013;54:1293-6. [Crossref] [PubMed]
- Moll G, Hult A, von Bahr L, et al. Do ABO blood group antigens hamper the therapeutic efficacy of mesenchymal stromal cells? PLoS One 2014;9:e85040. [Crossref] [PubMed]
- Večerić-Haler Ž, Kojc N, Sever M, et al. Case Report: Capillary Leak Syndrome With Kidney Transplant Failure Following Autologous Mesenchymal Stem Cell Therapy. Front Med (Lausanne) 2021;8:708744. [Crossref] [PubMed]
- Kim JS, Lee JH, Kwon O, et al. Rapid deterioration of preexisting renal insufficiency after autologous mesenchymal stem cell therapy. Kidney Res Clin Pract 2017;36:200-4. [Crossref] [PubMed]
- Karthik L, Kumar G, Keswani T, et al. Protease inhibitors from marine actinobacteria as a potential source for antimalarial compound. PLoS One 2014;9:e90972. [Crossref] [PubMed]
- Centeno CJ, Al-Sayegh H, Freeman MD, et al. A multi-center analysis of adverse events among two thousand, three hundred and seventy two adult patients undergoing adult autologous stem cell therapy for orthopaedic conditions. Int Orthop 2016;40:1755-65. [Crossref] [PubMed]
- Jiang W, Xu J. Immune modulation by mesenchymal stem cells. Cell Prolif 2020;53:e12712. [Crossref] [PubMed]
- Forslöw U, Blennow O, LeBlanc K, et al. Treatment with mesenchymal stromal cells is a risk factor for pneumonia-related death after allogeneic hematopoietic stem cell transplantation. Eur J Haematol 2012;89:220-7. [Crossref] [PubMed]
- Tootee A, Nikbin B, Nasli Esfahani E, et al. Clinical Outcomes of Fetal Stem Cell Transplantation in Type 1 Diabetes Are Related to Alternations to Different Lymphocyte Populations. Med J Islam Repub Iran 2022;36:34. [Crossref] [PubMed]
- Meng F, Xu R, Wang S, et al. Human umbilical cord-derived mesenchymal stem cell therapy in patients with COVID-19: a phase 1 clinical trial. Signal Transduct Target Ther 2020;5:172. [Crossref] [PubMed]
- Zhang J, Lv S, Liu X, et al. Umbilical Cord Mesenchymal Stem Cell Treatment for Crohn's Disease: A Randomized Controlled Clinical Trial. Gut Liver 2018;12:73-8. [Crossref] [PubMed]
- Harris VK, Stark J, Vyshkina T, et al. Phase I Trial of Intrathecal Mesenchymal Stem Cell-derived Neural Progenitors in Progressive Multiple Sclerosis. EBioMedicine 2018;29:23-30. [Crossref] [PubMed]
- Lin BL, Chen JF, Qiu WH, et al. Allogeneic bone marrow-derived mesenchymal stromal cells for hepatitis B virus-related acute-on-chronic liver failure: A randomized controlled trial. Hepatology 2017;66:209-19. [Crossref] [PubMed]
- Lalu MM, McIntyre L, Pugliese C, et al. Safety of cell therapy with mesenchymal stromal cells (MSCs): a systematic review. Am J Respir Crit Care Med 2010;181:A6043.
- Khalifeh Soltani S, Forogh B, Ahmadbeigi N, et al. Safety and efficacy of allogenic placental mesenchymal stem cells for treating knee osteoarthritis: a pilot study. Cytotherapy 2019;21:54-63. [Crossref] [PubMed]
- Ahn JO, Coh YR, Lee HW, et al. Human adipose tissue-derived mesenchymal stem cells inhibit melanoma growth in vitro and in vivo. Anticancer Res 2015;35:159-68. [PubMed]
- Zhang J, Hou L, Zhao D, et al. Inhibitory effect and mechanism of mesenchymal stem cells on melanoma cells. Clin Transl Oncol 2017;19:1358-74. [Crossref] [PubMed]
- Ning H, Yang F, Jiang M, et al. The correlation between cotransplantation of mesenchymal stem cells and higher recurrence rate in hematologic malignancy patients: outcome of a pilot clinical study. Leukemia 2008;22:593-9. [Crossref] [PubMed]
- Suzuki K, Sun R, Origuchi M, et al. Mesenchymal stromal cells promote tumor growth through the enhancement of neovascularization. Mol Med 2011;17:579-87. [Crossref] [PubMed]
- Uccelli A, Pistoia V, Moretta L. Mesenchymal stem cells: a new strategy for immunosuppression? Trends Immunol 2007;28:219-26. [Crossref] [PubMed]
- Soper SA, Rasooly A. Cancer: a global concern that demands new detection technologies. Analyst 2016;141:367-70. [Crossref] [PubMed]
- Yoon YS, Park JS, Tkebuchava T, et al. Unexpected severe calcification after transplantation of bone marrow cells in acute myocardial infarction. Circulation 2004;109:3154-7. [Crossref] [PubMed]
- Liao J, Chen X, Li Y, et al. Transfer of bone-marrow-derived mesenchymal stem cells influences vascular remodeling and calcification after balloon injury in hyperlipidemic rats. J Biomed Biotechnol 2012;2012:165296. [Crossref] [PubMed]
- Ge L, Xun C, Li W, et al. Extracellular vesicles derived from hypoxia-preconditioned olfactory mucosa mesenchymal stem cells enhance angiogenesis via miR-612. J Nanobiotechnology 2021;19:380. [Crossref] [PubMed]
- Rosová I, Dao M, Capoccia B, et al. Hypoxic preconditioning results in increased motility and improved therapeutic potential of human mesenchymal stem cells. Stem Cells 2008;26:2173-82. [Crossref] [PubMed]
- Yu X, Lu C, Liu H, et al. Hypoxic preconditioning with cobalt of bone marrow mesenchymal stem cells improves cell migration and enhances therapy for treatment of ischemic acute kidney injury. PLoS One 2013;8:e62703. [Crossref] [PubMed]
- Boyette LB, Creasey OA, Guzik L, et al. Human bone marrow-derived mesenchymal stem cells display enhanced clonogenicity but impaired differentiation with hypoxic preconditioning. Stem Cells Transl Med 2014;3:241-54. [Crossref] [PubMed]
- Hu C, Li L. Preconditioning influences mesenchymal stem cell properties in vitro and in vivo. J Cell Mol Med 2018;22:1428-42. [Crossref] [PubMed]
- Matthay MA, Calfee CS, Zhuo H, et al. Treatment with allogeneic mesenchymal stromal cells for moderate to severe acute respiratory distress syndrome (START study): a randomised phase 2a safety trial. Lancet Respir Med 2019;7:154-62. [Crossref] [PubMed]
- Riordan NH, Morales I, Fernández G, et al. Correction to: Clinical feasibility of umbilical cord tissue-derived mesenchymal stem cells in the treatment of multiple sclerosis. J Transl Med 2021;19:197. [Crossref] [PubMed]
- Bartolucci J, Verdugo FJ, González PL, et al. Safety and Efficacy of the Intravenous Infusion of Umbilical Cord Mesenchymal Stem Cells in Patients With Heart Failure: A Phase 1/2 Randomized Controlled Trial (RIMECARD Trial [Randomized Clinical Trial of Intravenous Infusion Umbilical Cord Mesenchymal Stem Cells on Cardiopathy]). Circ Res 2017;121:1192-204. [Crossref] [PubMed]
- Klabukov ID, Krasilnikova OA, Baranovskii DS. Quantitative human physiology: An introduction guide for advanced tissue engineering. Biotechnol J 2021; Epub ahead of print. [Crossref] [PubMed]
- Waters SL, Schumacher LJ, El Haj AJ. Regenerative medicine meets mathematical modelling: developing symbiotic relationships. NPJ Regen Med 2021;6:24. [Crossref] [PubMed]
- von Bahr L, Sundberg B, Lönnies L, et al. Long-term complications, immunologic effects, and role of passage for outcome in mesenchymal stromal cell therapy. Biol Blood Marrow Transplant 2012;18:557-64. [Crossref] [PubMed]
- Sedaghat A, Fazeli F, Jafari M, et al. Evaluation of the Immunomodulatory Effect of Mesenchymal Stem Cells on Sepsis-Induced Acute Respiratory Distress Syndrome: A Systematic Review. Int J Pediatr 2021;9:14105-15.
- Coppin L, Najimi M, Bodart J, et al. Clinical Protocol to Prevent Thrombogenic Effect of Liver-Derived Mesenchymal Cells for Cell-Based Therapies. Cells 2019;8:846. [Crossref] [PubMed]
- Caplan AI. Adult Mesenchymal Stem Cells: When, Where, and How. Stem Cells Int 2015;2015:628767. [Crossref] [PubMed]
- Kizilay Mancini Ö, Lora M, Shum-Tim D, et al. A Proinflammatory Secretome Mediates the Impaired Immunopotency of Human Mesenchymal Stromal Cells in Elderly Patients with Atherosclerosis. Stem Cells Transl Med 2017;6:1132-40. [Crossref] [PubMed]
- Krasilnikova OA, Klabukov ID, Baranovskii DS, et al. The new legal framework for minimally manipulated cells expands the possibilities for cell therapy in Russia. Cytotherapy 2021;23:754-5. [Crossref] [PubMed]
- Kuljanin M, Bell GI, Sherman SE, et al. Proteomic characterisation reveals active Wnt-signalling by human multipotent stromal cells as a key regulator of beta cell survival and proliferation. Diabetologia 2017;60:1987-98. [Crossref] [PubMed]
- Kordelas L, Rebmann V, Ludwig AK, et al. MSC-derived exosomes: a novel tool to treat therapy-refractory graft-versus-host disease. Leukemia 2014;28:970-3. [Crossref] [PubMed]
- Phinney DG, Pittenger MF. Concise Review: MSC-Derived Exosomes for Cell-Free Therapy. Stem Cells 2017;35:851-8. [Crossref] [PubMed]
- Chang CP, Chio CC, Cheong CU, et al. Hypoxic preconditioning enhances the therapeutic potential of the secretome from cultured human mesenchymal stem cells in experimental traumatic brain injury. Clin Sci (Lond) 2013;124:165-76. [Crossref] [PubMed]
Cite this article as: Baranovskii DS, Klabukov ID, Arguchinskaya NV, Yakimova AO, Kisel AA, Yatsenko EM, Ivanov SA, Shegay PV, Kaprin AD. Adverse events, side effects and complications in mesenchymal stromal cell-based therapies. Stem Cell Investig 2022;9:7.