Creation of human hematopoietic chimeric cell (HHCC) line as a novel strategy for tolerance induction in transplantation
Introduction
Vascularized composite allotransplantation (VCA) represents a new and promising reconstructive method for patients with severe, disfiguring injuries of the face, hands, abdominal wall, or groin region. However, in order to be effective and prevent allograft rejection, this new surgical approach requires lifelong immunosuppression which is responsible for severe side effects. Furthermore, standard immunosuppression protocols do not prevent chronic allograft rejection, which considerably limits routine application of VCA in the clinical scenario. Thus, new, more effective, and less toxic therapies have to be developed to support VCA and solid organ transplants.
Cell-based therapies including hematopoietic and mesenchymal stem cells (MSCs) are emerging as a new promising approach for tolerance induction and may reduce side effects of immunosuppression, due to their role in the alteration of local and systemic immune response of allograft recipients (1,2). In cases of VCA transplants supported with hematopoietic stem cells (HSCs) of donor origin (3) or adipose-derived stem cells (4,5), elevated levels of Foxp3+ regulatory T cells were detected facilitating induction of chimerism and tolerance.
Bone marrow transplantation (BMT) represents one of the well-established methods of tolerance induction by reprogramming the recipient’s immune system, to recognize the donor tissue as “self” (1,3,6,7). It was proven that induction of mixed hematopoietic chimerism prevents rejection and extends allograft survival (3,6,7). Reports on clinical cases of upper-extremity transplantation under the “Pittsburgh protocol” have demonstrated safety and tolerability of the bone marrow (BM) cell-based protocol allowing for reduced immunosuppression with low-dose of tacrolimus monotherapy (7). However, the toxicity of BMT protocols precludes this approach from routine clinical applications.
Our laboratory has over 20 years of research experience in chimerism induction and application of BM-based cell therapies in different VCA models (8-15). These pioneering studies on tolerance induction in VCA encouraged us to create the donor-recipient chimeric cells (DRCC) via ex vivo, polyethylene glycol (PEG)-mediated fusion.
This approach introduces a novel concept of cell fusion technology as a method of creating the new generation of DRCC to enhance engraftment, induce tolerance, and augment VCA survival without the need of life-long immunosuppression.
Based on the encouraging outcomes following application of chimeric cells (CC)-based protocols in different experimental models of VCA, we have created a new generation of BM-derived CD34+ human hematopoietic chimeric cells (HHCC) from the two unrelated human donors and tested in vitro the immunomodulatory and anti-inflammatory properties of HHCC as a potential supportive therapy for clinical cases of VCA and solid organ transplants.
Establishment of immunological tolerance remains the most important research goal of the transplantation field. Thus, introduction of HHCC therapy to the VCA transplants protocols will minimize the need for life-long immunosuppression and will contribute to induction of tolerance and as such will expand indications for face, hand, lower extremity, and abdominal wall transplants which will be directly applicable to the severely injured and disfigured patients. Moreover, HHCC therapy may be applied as a more effective therapeutic approach for patients suffering from a plethora of hematopoietic and immune deficiencies such as leukemia as well as the victims of radiation exposure. The advantages of the created donor-recipient HHCC include the reduced risk for development of graft-versus-host disease (GvHD), which introduces HHCC as a bridge therapy in BMT and as the rescue therapy in cases of acute post-radiation syndrome in military and civilian patients. The goal of this study was to assess in vitro the immunomodulatory and tolerogenic properties of the created HHCC representing a novel human chimeric cell line of hematopoietic lineage origin. First, we confirmed the stability and reproducibility of the HHCC creation via ex vivo, PEG-mediated fusion. Next, we characterized the phenotype, genotype and clonogenic properties of HHCC, whereas hematopoietic origin of HHCC was confirmed by expression of HSCs markers. Finally, immunomodulatory and tolerogenic potential of HHCC was confirmed by the mixed lymphocyte reaction (MLR) and increased expression of the pro-tolerogenic cytokines.
Introduction of HHCC as a new, BM-derived, cell-based therapy to the field of VCA represents a novel, regenerative medicine approach which will minimize the need for lifelong immunosuppression and will extend indications for VCA and solid organ transplants.
Methods
Isolation of human 34+ HSCs
Human hematopoietic CD34+ cells were isolated from human BM aspirates derived from two unrelated donors: a female and a male, and were purchased from Lonza Bioscience (Mapleton, IL, USA). Briefly, BM samples were diluted with three times the volume of PBS, transferred on Ficoll-Paque PLUS (Thermo Fisher Scientific, MA, USA) and centrifuged to separate BM components. The collected fraction of cells was suspended into the complete culture medium RPMI 1640 with 10% fetal bovine serum (FBS) (Thermo Fisher Scientific) and centrifuged. After centrifugation, the supernatant was removed and cells were washed with Dulbecco’s modified Eagle medium (DMEM) (Thermo Fisher Scientific) without serum and were centrifuged again. Further, supernatant was aspirated, and cells were suspended in Bead Buffer (Miltenyi Biotech, Germany). Next, CD34+ cells were magnetically labeled with CD34 MicroBeads UltraPure (Miltenyi Biotech). The cell fraction was loaded onto the MACS® Column (Miltenyi Biotech), placed in the magnetic field of a MACS® Separator (Miltenyi Biotech). Isolated CD34+ cells were cultured in vitro for up to 48 h in StemSpan™ H3000 medium (StemCell Technologies, Vancouver, Canada) supplemented with CD34+ expansion supplement StemSpan media (StemCell Technologies) and 10% of FBS (Thermo Fisher Scientific), the standard conditions to obtain a sufficient number of cells for fusion. The UIC Office for the Protection of Research Subjects has determined that this activity does not meet the definition of human subjects’ research as defined by 45 Code of Federal Regulations (CFR) 46.102(f). No ethical approval or informed consent is required because of the nature of this study.
Creation of HHCC via ex vivo PEG-mediated cell fusion procedure
The CD34+ cells were isolated from the BM harvested from two unrelated human donors (the male and the female donor) (Figure 1A). Next, the cells were washed in serum-free DMEM culture media (Gibco-ThermoFisher, MA, USA) supplemented with 1% Antibiotic-Antimycotic solution (Gibco-ThermoFisher) and counted in 0.4% Trypan Blue staining solution (Gibco-ThermoFisher). Then unstained parent CD34+ cells from the male and the female donor were collected for Paul Karl Horan dyes (PKH) staining, flow cytometry (FC), and electron microscopy (EM) analysis. Briefly, the pellet with the remaining parent cells was suspended in diluent C (Sigma, St. Louis, MO, USA) and 6 µL PKH dye was added into 2 mL of total volume. Each of the parent CD34+ cells were stained separately with PKH26 or PKH67 membrane tracking dye respectively according to the manufacturer’s instructions (Sigma). Next, the procedure was stopped with the addition of 1% bovine serum albumin (BSA). Prior to fusion, the cells were suspended in serum-free DMEM basal media, gently mixed and counted for viability in Trypan Blue. Cells were mixed in a ratio of 1:1. Further, the supernatant was removed, and PEG 4000 solution was slowly added (3.5 g of PEG) (EMD, Burlington, MA, USA). The fusion procedure was performed for 2 minutes. The fused cells were then washed in complete culture media (DMEM with 20% of FBS), samples for FC and confocal microscopy (CM) analysis were collected, then fused cells were centrifuged and suspended in DPBS-based fluorescence-activated cell sorting (FACS) buffer containing 25 mM HEPES, 2 mM EDTA and 1% FBS for FACS evaluation.
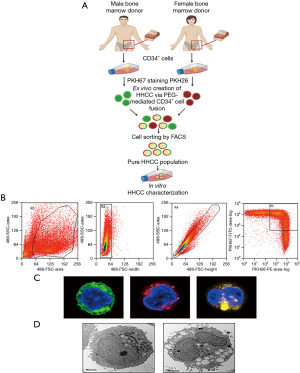
FACS analysis confirming HHCC fusion
Following the fusion procedure, the mix of two unfused PKH-labeled CD34+ parent cells was used to optimize sorter settings. Fusion of the CD34+ parent cells was analyzed to confirm the presence of double stained cells of yellow color (the merge of PKH67-green and PKH26-red). Double positive PKH26/PKH67 cells representing the fused human CD34+ chimeric cells from both donors, were sorted by the FACS (MoFlow Astrios, Beckman Coulter, CA, USA) and subjected to further analysis.
CM and EM analysis for confirmation of HHCC fusion
For CM of PKH labeled CD34+ parent cells of the male and female donors (controls) and the fused CD34+ cells (HHCC) were spun onto positively charged lysine coated microscope slides and mounted with VECTASHIELD® Antifade Mounting Medium with DAPI (Vector Laboratories, Burlingame, CA, USA). Cells were examined on XTCS-SP upright confocal microscope with Retiga 2000R camera (Leica, Wetzlar, Germany) and ImagePro Plus (Media Cybernetics, Rockville, MD, USA). EM analysis was performed to observe cell organelles in the CD34+ donor cells before fusion and in HHCC after cell fusion procedure, ×10,000 magnification was used (model 200-CX; JEOL USA, Electron Optics Div., Peabody, MA, USA).
Assessment of propagation and proliferation of HHCC
HHCC were propagated in H3000 (StemSpan media) with CD34+ expansion supplement (StemSpan media) (StemCell Technologies) and 10% of FBS (Thermo Fisher Scientific) in standard conditions. Proliferation properties of the CD34+ donor-derived cells, as well as the fused HHCC were assessed using CountBrightTM Absolute Counting Beads (Thermo Fisher Scientific). Briefly, cells were plated in a 24-well tissue culture plate (1×104 cells/well) and mixed with CountBrightTM absolute counting beads according to the manufacturer’s instructions. After adding the beads, cells were analyzed at days 0, 7, 14, 21 and 28 by FC (Gallios, Beckman Coulter) and the total number of cells was calculated based on the formula provided by the manufacturer. Kaluza software (Kaluza Analysis 2.1) was used to analyze the results.
Assessment of HHCC viability by Trypan Blue and LIVE/DEAD staining
Viability of CD34+ donor-derived cells, as well as the fused HHCC was determined by Trypan Blue (Gibco-ThermoFisher) and LIVE⁄DEAD® Fixable Dead Cell (Thermo Fisher Scientific) staining according to the manufacturer’s instructions at 3 hours and at 7-, 14- and 21-day post-fusion. The results were analyzed either by light microscopy (Leica, Wetzlar, Germany) for Trypan Blue staining or by FC for LIVE⁄DEAD analysis (Gallios, Beckman Coulter), Kaluza software (Kaluza Analysis 2.1) was used to analyze the results collected using the flow cytometer.
Assessment of HHCC apoptosis by Annexin V and TUNEL assays
To detect nuclear degradation and assess any signs of early apoptosis of CD34+ donor cells and the created HHCC, cells were stained with Annexin V-APC antibody (Thermo Fisher Scientific) and further analyzed by FC (Gallios, Beckman Coulter) at 3 hours, as well as at 4 and 7 days after fusion. Additionally, cells were stained with Sytox blue dye (Invitrogen, MA, USA) to separate dead cells from the apoptotic cells. For detection of nuclear degradation in the fused HHCC cells (late sign of apoptosis) TUNEL assay (Apo-BrdU™ TUNEL assay kit, Thermo Fisher Scientific) was performed at 3 hours and 7 days after cell fusion, according to the manufacturer’s instruction. The results were analyzed by FC (Gallios, Beckman Coulter), Kaluza software (Kaluza Analysis 2.1) was used to analyze the results.
Analysis of HHCC phenotype by FC
Characterization of HHCC hematopoietic cell surface markers was performed at 3- and 24-hour markers (CD34+, CD133+, CD117+, CD90+, CD4+, CD19+, CD14+) in the created HHCC. Moreover assessment of the following markers was evaluated for the expression of: the precursor stem cells (CD133+/CD90+), T-cells (CD4+/CD8+), T-regulatory cells (CD4+/CD25+), B-cells (CD5+/CD19+) and for the dendritic cells (CD123+/CD11c+) at 3 hours and 7 days of HHCC after the fusion. All cell stainings were performed in PBS containing 1% BSA with monoclonal antibodies, which were added at saturating concentrations. Incubation was performed for 30 min on ice, washed twice, resuspended in a staining buffer and analyzed using BD LSR Fortessa (Becton Dickinson, NJ, USA). For HHCC staining the following anti-human monoclonal antibodies were used: CD34 (APC, BD Biosciences, NJ, USA), CD133 (APC, Miltenyi Biotech), CD117 (APC, BD Biosciences), CD90 (BV421, BioLegend, CA, USA), CD4 (APC-Cy7, BD Biosciences), CD19 (APC-Cy7, BD Biosciences), CD19 (APC, BD Biosciences) and CD14 (APC, BD Biosciences), CD133+/CD90+ (APC, Miltenyi Biotech and BV421, BioLegend), CD4+/CD8+ (APC-Cy7, Miltenyi Biotech and BV421, BioLegend), CD4+/CD25+ (APC-Cy7, Miltenyi Biotech and BV421, BioLegend), CD5+/CD19+ (BV421, BioLegend and APC-Cy7, Miltenyi Biotech), and dendritic cells CD123+/CD11c+ (APC, Miltenyi Biotech and BV421, BioLegend). All samples were analyzed by FC (Gallios, Beckman Coulter). Kaluza software (Kaluza Analysis 2.1) was used to determine the phenotype of HHCC.
Assessment of HHCC polyploidy by fluorescence in situ hybridization (FISH)
To assess ploidy of CD34+ donors cells and HHCC, the standard FISH was performed at 3 hours after fusion. Briefly, cells were stained with specific X- and Y-chromosome painting probes (Human IDetect™ Chr X Paint Probe Red, Human IDetect™ Chr Y Paint Probe Green). Then, the slides were counterstained with DAPI and analyzed using a confocal microscope with Retiga 2000R camera (Leica, Wetzlar, Germany). The presence of at least three X- and one Y-chromosomes in HHCC was considered a polyploidy. The percentage of polyploid cells was determined based on the analysis of 1,250 cells.
Characterization of genotype of the created HHCC by polymerase chain reaction-reverse sequence-specific oligonucleotide probe (PCR-rSSOP) and short tandem repeats-polymerase chain reaction (STR-PCR)
PCR-rSSOP was used for HLA classes I and II typing to assess if the fused HHCC cells share antigens specific for both BM donors was performed using The DNA samples of donors CD34+ cells and HHCC were typed for HLA-A, -B, -Cw, -Bw, -DR, -DQ, -DR52/DR53 detection using commercial kits (LABtype rSSO Typing Test, OLI). DNA isolation was performed with DNeasy Blood and Tissue Isolation kit (Qiagen, Hilden, Germany) according to the manufacturer’s instructions. The DNA sample was subjected to PCR amplification (PE9700, Thermo Cycler Life Technologies, CA, USA) in a 10 µL reaction volume, with the PCR run at 96 °C for 3 min, 96 °C for 20 s, 60 °C for 20 s, and 72 °C for 20 s, for 5 cycles, and 96 °C for 10 s, 60 °C for 15 s, and 72 °C for 20 s for 30 cycles followed by 72 °C for 10 min and stored at 4 °C forever. After amplification, the PCR products were denatured and neutralized with acids and bases and hybridized with the corresponding locus beads at 60 °C for 15 min, which were washed three times using the washing buffer. Further, the products were subjected to a reaction with streptavidin conjugated phycoerythrin (SAPE) for 5 min at 60 °C, then washed and suspended with 60 µL of washing buffer. The fluorescence signals of the products were identified by the laser Luminex 200 (Luminex, TX, USA). Lastly, the HLA typing was obtained from the software HLAtools. Further the STR-PCR analysis was performed to determine the presence of all short tandem repeat’s loci specific for both fusion donors in the HHCC population at 3 hours and at 7 days post-fusion. Genetic fingerprinting was performed by STR-PCR using Promega’s GenePrint 10 kit following manufacturer’s protocol (Promega Corporation, Madison, WI, USA) at 3 hours and at 7 days after fusion. Genomic DNA was extracted from two donors and HHCC cells pellets using the Maxwell® 16 Tissue DNA purification kit (Promega Corporation). One pellet per sample was transferred to well #1 of the cartridges and the cell extraction protocol was selected to run according to the manufacturer’s instructions. The extracted genomic DNA was quantified using Qubit®2.0 Fluorometer (Thermo Fisher Scientific) and assessed for the DNA quality using Agilent’s TapeStation (Agilent, CA, USA) instrument. Each sample was composed of 1 µL of genomic DNA at a concentration of 5 ng/µL, 5 µL of Master Mix, 5 µL Primer Pair Mix, and 14 µL of amplification grade water. In addition, positive and negative control samples were prepared in conjunction with the samples. PCR was conducted using the following thermal conditions: 96 °C for 1 min, followed by 30 cycles of 94 °C for 10 s, 59 °C for 1 min, 72 °C for 30 s; a subsequent temperature step at 60 °C for 10 min and a final storage step at 4 °C. Once amplified, each sample was prepared for capillary electrophoretic analysis using 1 µL of the PCR amplified reaction, 1 µL of internal ladder provided by the commercial kit, and 9 µL of HiDi formamide. Data was obtained for the following loci-genetic markers: TH01 D5S818, D21S11, D7S820, CSF1PO, AMEL (amelogenin), and TPOX and was reported in the tabulated form for analysis.
Assessment of clonogenic properties of HHCC by colony forming unit (CFU)
To assess clonogenic properties the samples of the unstained CD34+ cells, PKH26 and PKH67 stained CD34+ cells and fused (HHCC) were collected and analyzed at 3 hours and 7 days after fusion. Briefly, cells were cultured in separate flasks, 1×103 cells were plated in a 35 mm dishes following the MethoCult® (StemCell Technologies) manufacturer’s instructions in optimized methylcellulose-based medium (MethoCult® H4034 StemCell Technologies) supporting the optimal growth of the erythroid progenitors [CFU-E and burst forming unit (BFU)-E], granulocyte macrophage progenitors (CFU-GM, CFU-G and CFU-M) and the multi-potential granulocyte, erythroid, macrophage, megakaryocyte progenitors (CFU-GEMM). The cells were incubated for 3 hours and 7 days and then the number of colonies was scored under the light microscope (Leica DM 5500B Compound Microscope with Leica DFC290 Color Digital Camera, Leica, Germany).
Assessment of tolerogenic and immunomodulatory properties of HHCC by MLR and reverse transcription polymerase chain reaction (RT-PCR) for cytokine expression
Tolerogenic and immunomodulatory properties of HHCC were assessed using MLR assay. Donor peripheral T-cells (responders) were activated with a cell activation cocktail (BioLegend) stained with 3 µM CellTrace™ Violet Cell Proliferation Dye (BD Biosciences) and plated in the density of 2.5×105/well in a 96-well plate. Freshly fused HHCC (3 h after fusion) or T-cells from unmatched donors were inactivated by irradiation (30 Gy) using a 137Cs source (Gamma cell® 3000, ON, Canada) and added to the T-cells-responders in ratio 1:1. Mixed responder and stimulator cells were cultured in 200 µL of “complete” RPMI 1640 medium containing 10% FBA and 1X antibiotic/antimycotic solution (1XAAS, Thermo Fisher Scientific) for 4 days. At 4 days after cell plating and culturing, the cells were harvested and stained with mouse anti-human CD8 antibody (BD Bioscience). T-cell proliferation was measured by decreased fluorescent dye intensity by FC (Gallios, Beckman Coulter).
The secreting properties and cytokine profile of pro-tolerogenic Th2 [interleukin (IL)-10, transforming growth factor-β (TGF-β)] and pro-inflammatory Th1 [tumor necrosis factor-α (TNF-α)] cytokines of created HHCC was assessed by real-time PCR. Total RNA was isolated from unfused CD34+ donor cells before fusion and from fused HHCC at 3 hours, 7 days and 14 days after fusion with the RNeasy kit (Qiagen) following the manufacturer’s instructions. Next, the RNA was reverse transcribed with High-Capacity cDNA Reverse Transcription kit (Applied Biosystems, MA, USA) and analyzed on an ABI 7500 instrument. Real-time conditions were as follows: 95 °C (15 s), 40 cycles at 95 °C (15 s), and 60 °C (1 min). The relative quantity of a target was normalized to the endogenous GAPDH gene as control and relative to a calibrator (ABI Prism 7500 software).
Statistical analysis
Data are presented as mean ± standard deviation. OriginPro 2017 was used for statistical analyses. Differences between respective groups were assessed using Student’s t-test. P values were considered significant below 0.05.
Results
Confirmation of creation of HHCC line via PEG-mediated cell fusion
The experimental design of ex vivo PEG mediated cell fusion of the human CD34+ cells derived from two unrelated (the male and the female) BM donors is presented on Figure 1A. After fusion, the population of 60.21% of fused CD34+ cells were further analyzed and sorted by FACS (Figure 1B, left image, gate R1). PKH staining assessed by FACS confirmed staining efficacy for PKH67 at 99.18% (Figure 1B, middle image, gate R2) and for PKH26 at 91% (Figure 1B, middle image, gate R4). Fusion efficacy revealed 48.29% (Figure 1B, right image, gate R3). Post-fusion procedure, the created HHCC cells were analyzed by CM (Figure 1C). The male donor CD34+ cells were stained with PKH67 (green) and the female donor CD34+ cells were stained with PKH26 (red). Fusion of the CD34+ parent cells was confirmed by the presence of the double stained PKH67/PKH26 cells of orange/yellow color (the merge of PKH67-green and PKH26-red). The EM analysis allowed detection of single CD34+ cells before fusion with a single nucleus and presence of two nuclei in the HHCC after cell fusion (Figure 1D). We confirmed successful fusion of the CD34+ cells from two unrelated donors and the creation of a new line of hematopoietic HHCC was confirmed by CM, FACS analysis and EM.
Confirmation of in vitro propagation of HHCC after cell fusion
Several media were tested to optimize the in vitro culturing conditions for the created HHCC. The best efficiency of cell propagation was achieved using the StemSpanTM H3000 (StemCell Technologies) medium supplemented with the StemSpanTM CD34+ expansion supplement. The images of expansion of the fused cells in the culture supported StemSpanTM H3000 medium over 28 days are shown in Figure 2A. The number of the propagating unfused CD34+ cells and fused HHCC cells was determined at 0, 7, 14, 21 and 28 days after culturing using CountBrightTM absolute counting beads for unused CD34+ cells and fused CD34+ cells. The unfused CD34+ cells revealed rapidly increasing growth over time and the number increased over 100 times after 21 days of culturing followed by the cell number decrease at day 28 of cell culturing. HHCC cells showed more stable growth and negligible decrease between day 21 and 28 of cell culturing (Figure 2B).
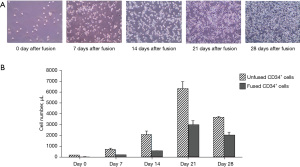
Confirmation of HHCC fusion safety by high viability and low apoptosis levels
Assessment of the viability of the created HHCC after fusion was performed by Trypan Blue staining (Figure 3A) and remained at 98.66% level over 21 days of HHCC culturing. Cell fusion did not affect HHCC viability (Figure 3B). Additionally LIVE/DEAD assay confirmed high viability of HHCC after cell fusion and revealed 1.34% of dead cells at 3 hours and 1.76% of dead cells at 7 days after fusion (Figure 3C). The apoptosis of HHCC was evaluated using the Annexin V staining (early stage of apoptosis), Sytox Blue (presence of dead cells) and TUNEL assay (late stage of apoptosis). Assessment of HHCC apoptosis after cell fusion showed: at 3 hours, 2.72% of cells in the early stage of apoptosis stained with Annexin V and 1.31% of dead cells stained with Sytox Blue, at day 4 after cell fusion—3.2% of cells in early stage of apoptosis (Annexin V) with low percentage of dead cells—0.57% (Sytox Blue) and at 7 days after fusion—2.52% cells in early stage of apoptosis (Annexin V) and 0.81% of dead cells (Sytox Blue) (Figure 3D). The TUNEL assay analysis confirmed high viability of HHCC (99%) and low levels of HHCC in late stage of apoptosis (1.23% at 3 hours after fusion and 2.19% at 7 days after fusion) (Figure 3E). Additionally, the percentage of the apoptotic HHCC cultured for up to 21 days was low and ranged between 3.2–3.9% (Figure 3F).
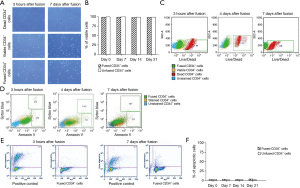
Confirmation of hematopoietic phenotype of the created HHCC
The phenotype assessment of HHCC at 3 and 24 hours after cell fusion was determined by FC and presented as histograms (Figure 4A). The ex vivo created HHCC expressed the following: hematopoietic and precursor stem cell markers summarized in Figure 4B: CD34+ reached 95.6% at 3 hours after fusion and declined to 91.6% at 24 hours after fusion. The level of stem cell/precursor marker of CD133+ was assessed at 0.71% at 3 hours after the fusion and increased to 2.11% at 24 hours post-fusion. The percentage of cells expressing CD19+, the B-cells marker, dropped from 6.11% at 3 hours after fusion to 4.75% during the 24-hour culturing. However, the level of CD4+ expression, a marker of T-cells, increased from 0.93% at 3 hours post-fusion to 1.79% at 24 hours post-fusion. No significant changes were observed in the levels of hematopoietic cell marker expressions after cell fusion, confirming stability of the phenotype of the created HHCC. Additionally, expression of T-regulatory cells markers (CD4+/CD25+) in the naive-donor CD34+ cells and HHCC assessed by FC after 7 and 21 days of cell culturing revealed 0.69% of positive HHCC cells after 7 days and 2.4% after 21 days of cell culturing. Representative dot-plots are presented in Figure 4C. Moreover, the level of the CD133+/CD90+ marker revealed at 2.3% at 3 hours after fusion and declined to 1.39% at 7-day post-fusion. However, the percentage of CD4+/CD8+, CD4+/CD25+, CD5+/CD19+, and CD123+/CD11c+ cell markers was higher in the HHCC population after 7 days of culturing when compared with values assessed at 3 hours after cell fusion, indicating the potential tolerogenic properties of HHCC cells (Figure 4D). No significant changes were observed between CD markers levels at 3 hours and 7 days after cell fusion.
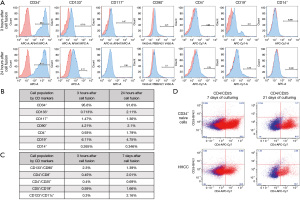
Confirmation of low level of polyploidy and the created HHCC
Standard FISH of polyploidy for male (XY) and female donors (XX) (Figure 5A) before fusion as well as for HHCC (Figure 5B) after fusion revealed a low level of 0.48% of the polyploid cells in the HHCC population at 3 hours after cell fusion.
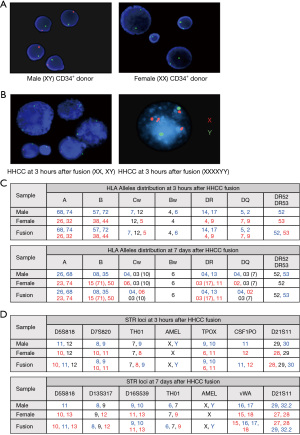
Confirmation of the donor specific genotype in the created HHCC
The HHCC assessment by PCR-rSSOP confirmed presence of the HLA antigens (A, B, Cw, Bw, DR, DQ, DR52 and DR53) specific for both CD34+ cell donors (the male and the female) at 3 hours, as well as at 7 days after cell fusion (Figure 5C). The STR-PCR assessment of HHCC confirmed presence of the short tandem repeat’s loci specific for both fusion donors (the male and the female) at 3 hours and 7 days after cell fusion (Figure 5D).
Confirmation of clonogenic properties of the created HHCC
Clonogenic properties of HHCC at 3 hours after fusion and at 7 days of cell culturing were evaluated by a CFU assay. Representative images of the created colonies of CFU-GM, BFU-E and CFU-GEMM are presented on Figure 6A. As shown on Figure 6B, the created HHCC has clonogenic potential and differentiates into all classes of myeloid and erythroid progenitor cells. Assessment of culture of the unfused, PKH stained CD34+ donor cells and the fused CD34+ chimeric cells (HHCC) for up to 7 days revealed a decreased number of the CFU-GM, BFU-E and CFU-GEMM colonies.
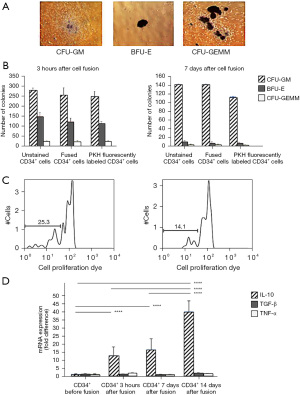
Confirmation of immunomodulatory and tolerogenic properties of the created HHCC
Assessment of immunogenic properties of HHCC by a one-way MLR assay revealed lower (14.1%) response to HHCC compared to controls of the 3rd party allogenic T-cells (25.3%) after 4 days of cell culture. This confirmed decreased immunogenicity of HHCC and thus, pro-tolerogenic profile of the HHCC cell line (Figure 6C). Moreover, assessment of cultured HHCC performed by RT-PCR revealed significantly increased expression of the IL-10-the pro-tolerogenic Th2 cytokine at 3 hours, 7 days and 14 days after fusion when compared to the levels assessed before cell fusion P<0.0001 (Figure 6D). There was no change observed in the expression of the proinflammatory Th1 cytokine (TNF-α) and protolerogenic Th2 cytokine (TGF-β) over 14 days of culture of unfused CD34+ donor cell controls and the fused HHCC (Figure 6D).
Discussion
VCA is the most promising reconstructive solution that provides satisfactory outcomes for civilian and military patients suffering from massive tissue loss. However, the need to support VCA with life-long immunosuppression to avoid rejection is the major obstacle precluding routine applications of VCA in clinical practice.
Therefore, tolerance induction and elimination of lifelong toxic immunosuppressive regimens preventing allograft rejection have been the major focus of the VCA research. Tolerance induction achieved by establishing mixed hematopoietic chimerism through HSCs transplantation is considered one of the most promising approaches (16,17). Studies on combined kidney and bone marrow transplantation (CKBMT) have confirmed donor specific chimerism in kidney allograft recipients, which allowed for tapering of the immunosuppressive management (16-18). Immunosuppressive regimens currently used in clinical cases of VCA are based on the protocols established for solid organ transplants. Although these protocols extend allograft survival rates, the occurrence of acute allograft rejection is still high and varies between different VCA transplants (7,19). Clinical VCA cases of hand and face transplantation introduced protocols of combined immunosuppression with BMT and CD34+ stem cells respectively (7,20).
However, strategies with BMT require recipient conditioning which is often associated with severe side effects including GvHD (6).
Another tolerance inducing protocol applied in VCA includes alemtuzumab with tapered steroids level followed by BM infusion on the post-transplant day 14 and maintenance of tacrolimus protocol (7). However, despite this promising approach, none of the hand transplant recipients under combined BMT and tacrolimus protocol have developed tolerance and continuation of lifelong immunosuppression was required, exposing patients to the immunosuppression induced severe side effects (7).
Therefore, current immunosuppressive protocols are toxic and none of the tested combined BMT/immunosuppressive protocols have led to tolerance induction. Thus, there is an urgent need to develop new, cell-based therapies, which will extend allograft survival without the need for harmful conditioning protocols or life-long immunosuppression. New stem cell strategies are based on the tailored, customized approaches to address the individual patient needs and to change the current paradigm that one standard immunosuppressive protocol “fits” all transplant patients irrespective of the age, gender, race and transplanted organ.
To address these limitations, for the past 20 years, our Laboratory has developed novel protocols for induction of tolerance via establishment of donor specific chimerism tested in different experimental models of VCA, including limb allograft transplant carrying vascularized BM component (8-15). Based on this experience confirming a crucial role of the vascularized BM component of donor origin in induction of donor specific chimerism, we have created DRCC via PEG-mediated fusion of BM cells derived from two unrelated donors (13). The tolerogenic potential of DRCC was confirmed in vivo by prolonged VCA survival and induction of mixed chimerism (13). Thus, in the search of new, clinically applicable alternative approaches to BMT, we have created a new generation of human donor-derived chimeric cells (DCC) via ex vivo cell mediated fusion of BM-derived CD34+ cells and/or MSCs from two unrelated human donors. We have successfully confirmed tolerogenic properties of DCC in the well-established models of the VCA and heterotopic heart transplants, where long-term survival (100 days) was achieved in the heart and VCA transplant recipients supported with DCC therapy of CD34+ and/or MSCs origin (manuscript in preparation). We anticipate that creation of chimeric cells of MSCs origin may exert a more significant tolerogenic effect due to well-known immunomodulatory properties of MSCs.
Based on the encouraging results of DCC therapy, the goal of the current study was to confirm reproducibility of human CD34+ stem cell fusion as well as to assess in vitro the immunomodulatory and tolerogenic properties of a new generation of HHCC line as a potential-clinically applicable therapy in the field of VCA and solid organ transplants. We have confirmed stability and reproducibility of the PEG-mediated fusion of the human CD34+ HSCs derived from the two unrelated donors. After fusion the genotype of the created HHCC was confirmed by presence of the HLA class I and class II markers and STR loci specific for both, CD34+ donors. Moreover, FISH analysis confirmed a negligible number of the polyploid cells, further supporting safety of cell fusion protocol. Finally, the hematopoietic origin of the created HHCC was confirmed by expression of the HSCs surface markers.
In order to consider potential clinical applications of HHCC, we have characterized in vitro the propagative and proliferative properties of the HHCC confirming normal proliferation of the fused HHCC which was comparable with proliferation rates of the unfused-control human CD34+ cells grown in the standard HSCs culture media and conditions.
High viability of HHCC after fusion was maintained over a 21-day of evaluation period and was comparable to viability of the unfused CD34+ cells. Moreover, the low rate of both the early and late stages of HHCC apoptosis was confirmed by the TUNEL assay and the Annexin V assay further confirming stability of the created HHCC cell line.
The clonogenic properties were confirmed by differentiation of HHCC into the myeloid and erythroid progenitor cells, further verifying proliferative properties of HHCC.
The mechanism of action of the created HHCC is based on the genetic match between the donor and recipient after ex-vivo PEG-mediated fusion procedure of the CD34+ cells derived from the two unrelated human BM donors. As a result, the created donor-recipient chimeras are caring approximately 50% of the HLA class I and class II alleles and 50% of the STR loci from each of the BM donor as confirmed by PCR-rSSOP and STR-PCR analysis respectively. Thus, following fusion of the CD34+ cells, the created HHCC represent the haploidentical match between the donor and the recipient which reduces the immune response and enhances tolerogenic potential of HHCC as confirmed in our study by MLR revealing decreased immune response of the HHCC. In this context, HHCC will support allograft engraftment with reduced or limited need for life-long immunosuppression.
In addition, the increased expression of the pro-tolerogenic cytokine IL-10 and negligible expression of the proinflammatory Th1 cytokine (TNF-α) further confirmed immunomodulatory properties and tolerogenic potential of the created HHCC cell line. These findings are in line with our previous experimental studies confirming the tolerogenic effect of chimeric cells used to support different VCA transplants without immunosuppression protocol (13,15).
Results of our study confirmed stability of the HHCC phenotype, as revealed by expression of the HSCs markers and increased expression of T regulatory cells markers (CD4/CD25) further supporting immunomodulatory as well as tolerogenic properties of HHCC therapy.
In summary, in this study, we have confirmed the reproducibility and genetic stability of the created new generation of human HHCC cell line of hematopoietic lineage origin and have confirmed both the efficacy and safety of cell fusion procedure. In addition, clonogenic differentiation and propagation properties of HHCC performed under standard ex vivo cell culture conditions were confirmed. Currently, there are no immunomodulatory or tolerogenic cell-based therapies available for tolerance induction in VCA and solid organ transplants. Thus, introduction of a new BM-derived, HHCC cell-based therapy to the field of VCA, represents a novel, regenerative medicine approach which will minimize or reduce the need for lifelong immunosuppression and will expand clinical indications for VCA transplants including face, hand, lower extremity, abdominal wall as well as solid organ transplants. Based on the encouraging results of the in vitro characterization of the created HHCC cell line outlined in this study, as a next step in the development of HHCC therapy for potential clinical applications we are planning to test immunomodulatory properties as well as efficacy and safety of HHCC in vivo, in the athymic homozygous nude rat model, which allows to study engraftment, migration and biodistribution of HHCC of the human origin.
This will open broad application of VCA to severely disfigured patients suffering from motor-vehicle accidents and battlefield-related injuries, and as such will change the current paradigm of reconstructive transplantation and will have significant impact on the quality of life of the VCA transplant recipients.
To the best of our knowledge, this is the first report on the successful creation of the new human hematopoietic cell line of HHCC via ev vivo PEG-mediated fusion of the human CD34+ cells derived from the two unrelated donors.
Conclusions
This study confirmed feasibility and reproducibility of creation of a novel HHCC line from the two unrelated BM donors. We have successfully characterized HHCC viability, phenotype, genotype and the immunomodulatory and tolerogenic properties of HHCC. Therefore, HHCC represents a novel cell-based therapeutic strategy for chimerism and tolerance induction in the VCA and solid organs transplantation.
Acknowledgments
The authors thank Ewa Bryndza, PhD, Katarzyna Futoma, PhD, and Joanna Cwykiel, PhD, for technical support and staff members of Core Imaging Facilities at University of Illinois at Chicago for help with acquiring confocal microscopy images, and Flow Cytometry Core for technical assistance with HHCC sorting and acquisition of flow cytometry data.
Funding: This work was supported by the Army, Navy, NIH, Air Force, VA and Health Affairs to support the AFIRM II effort under Award No. W81XWH-13-2-0053, and The U.S. Army Medical Research Acquisition Activity, 820 Chandler Street, Fort Detrick MD 21702-5014.
Footnote
Conflicts of Interest: All authors have completed the ICMJE uniform disclosure form (available at https://sci.amegroups.com/article/view/10.21037/sci-2022-026/coif). MS reports consulting fees from AMCA. The other authors have no conflicts of interest to declare.
Ethical Statement: The authors are accountable for all aspects of the work in ensuring that questions related to the accuracy or integrity of any part of the work are appropriately investigated and resolved. The UIC Office for the Protection of Research Subjects has determined that this activity does not meet the definition of human subjects’ research as defined by 45 CFR 46.102(f). No ethical approval or informed consent is required because of the nature of this study.
Open Access Statement: This is an Open Access article distributed in accordance with the Creative Commons Attribution-NonCommercial-NoDerivs 4.0 International License (CC BY-NC-ND 4.0), which permits the non-commercial replication and distribution of the article with the strict proviso that no changes or edits are made and the original work is properly cited (including links to both the formal publication through the relevant DOI and the license). See: https://creativecommons.org/licenses/by-nc-nd/4.0/.
References
- Zuber J, Sykes M. Mechanisms of Mixed Chimerism-Based Transplant Tolerance. Trends Immunol 2017;38:829-43. [Crossref] [PubMed]
- Pilat N, Farkas AM, Mahr B, et al. T-regulatory cell treatment prevents chronic rejection of heart allografts in a murine mixed chimerism model. J Heart Lung Transplant 2014;33:429-37. [Crossref] [PubMed]
- Mathes DW, Chang J, Hwang B, et al. Simultaneous transplantation of hematopoietic stem cells and a vascularized composite allograft leads to tolerance. Transplantation 2014;98:131-8. [Crossref] [PubMed]
- Kuo YR, Chen CC, Goto S, et al. Modulation of immune response and T-cell regulation by donor adipose-derived stem cells in a rodent hind-limb allotransplant model. Plast Reconstr Surg 2011;128:661e-72e. [Crossref] [PubMed]
- Pappalardo M, Montesano L, Toia F, et al. Immunomodulation in Vascularized Composite Allotransplantation: What Is the Role for Adipose-Derived Stem Cells? Ann Plast Surg 2019;82:245-51. [Crossref] [PubMed]
- Thaiss CC, Oura T, Sasaki H, et al. Importance of Hematopoietic Mixed Chimerism for Induction of Renal Allograft Tolerance in Nonhuman Primates. Transplantation 2019;103:689-97. [Crossref] [PubMed]
- Schneeberger S, Gorantla VS, Brandacher G, et al. Upper-extremity transplantation using a cell-based protocol to minimize immunosuppression. Ann Surg 2013;257:345-51. [Crossref] [PubMed]
- Siemionow M. Impact of reconstructive transplantation on the future of plastic and reconstructive surgery. Clin Plast Surg 2012;39:425-34. [Crossref] [PubMed]
- Siemionow M, Klimczak A. Chimerism-based experimental models for tolerance induction in vascularized composite allografts: Cleveland clinic research experience. Clin Dev Immunol 2013;2013:831410. [Crossref] [PubMed]
- Siemionow M, Rampazzo A, Gharb BB, et al. The reversed paradigm of chimerism induction: Donor conditioning with recipient-derived bone marrow cells as a novel approach for tolerance induction in vascularized composite allotransplantation. Microsurgery 2016;36:676-83. [Crossref] [PubMed]
- Siemionow M, Cwykiel J, Heydemann A, et al. Creation of Dystrophin Expressing Chimeric Cells of Myoblast Origin as a Novel Stem Cell Based Therapy for Duchenne Muscular Dystrophy. Stem Cell Rev Rep 2018;14:189-99. [Crossref] [PubMed]
- Zor F, Bozkurt M, Cwykiel J, et al. The effect of thymus transplantation on donor-specific chimerism in the rat model of composite osseomusculocutaneous sternum, ribs, thymus, pectoralis muscles, and skin allotransplantation. Microsurgery 2020;40:576-84. [Crossref] [PubMed]
- Cwykiel J, Jundzill A, Klimczak A, et al. Donor Recipient Chimeric Cells Induce Chimerism and Extend Survival of Vascularized Composite Allografts. Arch Immunol Ther Exp (Warsz) 2021;69:13. [Crossref] [PubMed]
- Siemionow M, Ortak T, Izycki D, et al. Induction of tolerance in composite-tissue allografts. Transplantation 2002;74:1211-7. [Crossref] [PubMed]
- Hivelin M, Klimczak A, Cwykiel J, et al. Immunomodulatory Effects of Different Cellular Therapies of Bone Marrow Origin on Chimerism Induction and Maintenance Across MHC Barriers in a Face Allotransplantation Model. Arch Immunol Ther Exp (Warsz) 2016;64:299-310. [Crossref] [PubMed]
- Scandling JD, Busque S, Dejbakhsh-Jones S, et al. Tolerance and chimerism after renal and hematopoietic-cell transplantation. N Engl J Med 2008;358:362-8. [Crossref] [PubMed]
- Scandling JD, Busque S, Shizuru JA, et al. Chimerism, graft survival, and withdrawal of immunosuppressive drugs in HLA matched and mismatched patients after living donor kidney and hematopoietic cell transplantation. Am J Transplant 2015;15:695-704. [Crossref] [PubMed]
- Lee KW, Park JB, Park H, et al. Inducing Transient Mixed Chimerism for Allograft Survival Without Maintenance Immunosuppression With Combined Kidney and Bone Marrow Transplantation: Protocol Optimization. Transplantation 2020;104:1472-82. [Crossref] [PubMed]
- Kueckelhaus M, Fischer S, Seyda M, et al. Vascularized composite allotransplantation: current standards and novel approaches to prevent acute rejection and chronic allograft deterioration. Transpl Int 2016;29:655-62. [Crossref] [PubMed]
- Dubernard JM, Devauchelle B. Face transplantation. Lancet 2008;372:603-4. [Crossref] [PubMed]
Cite this article as: Siemionow M, Brodowska S, Różczka K, Roesler C. Creation of human hematopoietic chimeric cell (HHCC) line as a novel strategy for tolerance induction in transplantation. Stem Cell Investig 2022;9:11.