Shedding light on HSC dormancy—a role for the DARC
Hematopoietic stem cell (HSC) quiescence is vital for lifelong hematopoiesis as it maintains long-term potential and self-renewal capacity of HSCs. Hypoxia is one feature of the bone marrow (BM) microenvironment thought to contribute to quiescence by limiting oxidative stress and initiating a distinct metabolic program (1). Based on their prior identification of a hypoxia response element in the promoter region of the tetraspanin CD82 gene (2), Hur and colleagues sought to address the role of this molecule in HSC function (3). Consistent with hypoxia inducible factor 1 (HIF-1α) expression (4), long term-HSCs (LT-HSCs) were found to be the exclusive primitive hematopoietic cell type expressing CD82. Expression of CD82 initiated a dormancy-enforcing program dependent upon TGFβ signaling. Furthermore, CD82 expression on HSCs was maintained by a subset of macrophages (MΦs), an emerging niche cell for HSCs. Hematopoietic demands that occur as a result of injury or infection must interrupt dormancy-enforcing programs. As MΦs are important immune sentinels, the study suggests MΦs may directly control HSC function in demand-adapted hematopoiesis and in conditions of acute or chronic inflammation (5). Thus, the insights provided by Hur et al. have broad implications for understanding HSC function in demand-adapted hematopoiesis, as well as aging and cancer.
Novel marker of HSC quiescence
Though numerous phenotypic markers for HCSs exist, few are linked directly to a functional attribute, such as dormancy. The observation that CD82 expression was exclusive to the pool of LT-HSCs in both mice and humans, and was linked functionally to reduced HSC cycling, indicates its use for identifying quiescent HSCs. CD82 (also referred to as KAI1) is a member of the family of tetraspanins, a highly conserved family of integral membrane proteins involved in cell adhesion, membrane trafficking, and signaling [reviewed in (6)]. Tetraspanins can both promote and suppress cancer metastases owing to their ability to modulate proliferation, migration, and senescence. CD82 was found to suppress metastases via its interaction with Duffy antigen receptor for chemokines (DARC), present on vascular endothelium (7). Indeed, solid tumors that lack CD82 expression have been shown to be more metastatic and proliferative (8,9). HSC quiescence does not absolutely require CD82, however, as a portion of HSCs remains in G0 in the absence of CD82, and mice lacking CD82 do not exhibit signs of hematopoietic dysfunction even at 18 months (10). In fact, only about one-third of the pool of phenotypic LT-HSCs (or SLAM-HSCs) expresses CD82. It will be interesting to compare CD82+ and CD82− HSCs from wild-type mice to determine whether a functional gradient exists among the HSC pool potentially regulated by CD82 expression. Nonetheless, in the absence of CD82, there was a specific reduction in LT-HSCs and an increased number of proliferating HSCs, while more differentiated progenitors were spared and comparable to wild type counterparts. So, how does CD82 enforce dormancy? To address this question a series of in vitro experiments was performed utilizing a progenitor cell line wherein CD82 was either overexpressed or knocked down. Cell cycle dependent kinase inhibitors were reduced upon CD82 knockdown, and expression of TGFβ signaling components, TGFβ1 and TGFβR2, were diminished by both gene expression and protein. CD82-mediated TGFβ1 expression, and maintenance of G0, was inhibited by blocking PKCα, a molecule previously shown to associate with CD82 (11). In line with the function of CD82 in promoting LT-HSC dormancy, the tetraspanin CD81 is necessary for preserving HSCs under myeloablative stress by promoting reentry into dormancy upon proliferation (12).
CD82-mediated maintenance of dormancy is clinically relevant as dormancy enhances homing and engraftment of HSCs in BM transplantation, a life-saving treatment for a variety of hematopoietic diseases. It is interesting to note that HSC proliferation impedes homing and engraftment in part due to altered membrane structures and a loss of membrane polarization (13). In fact, CD82-enriched polarized membrane domains are critical for engagement with the BM microenvironment, a first step in engraftment. Thus, CD82 expression may not only enforce dormancy but also create a physical platform necessary for engraftment. CD82 increases the molecular density of α4β1 (very late antigen or VLA-4) (14), which augments binding/adhesion to osteopontin and vascular adhesion molecule (VCAM-1) (15,16), improving transplantation efficiency. As α4β1 controls homing and mobilization (17,18), one possible explanation for reduced BM HSCs in CD82-deficient mice may be increased mobilization due to altered α4β1 clustering/expression. Altogether, regulation of cell cycle and matrix adherence by tetraspanins suggests these membrane proteins support hematopoiesis by enforcing the HSC dormancy program, and CD82 appears to play multiple roles that converge on maintaining dormant HSCs in the bone marrow.
Dormancy and self-renewal programs can be detrimental in the context of cancer, and tetraspanins have also been found to be upregulated on leukemic cells (19). CD82 is overexpressed in acute myelogenous leukemia, enhancing adhesion and possibly increasing homing and lodging in the bone marrow (20,21). CD82 expression also positively correlated with the survival of leukemic cells via the IL-10/STAT5 pathway (22) and via upregulation of antiapoptotic genes (23). Thus, in addition to enforcing dormancy, CD82 coordinates survival and homing cues. While these features are likely necessary for normal HSC function, they are exploited by leukemic stem cells, highlighting the therapeutic potential of targeting CD82 in leukemia.
The role of CD82 in HSC maintenance and function likely goes beyond an intrinsic role, and extends to the HSC niche. Hur et al. demonstrate an indirect impact of CD82 deficiency on the HSC microenvironment as mesenchymal stromal cells (MSCs) were found to express CD82 and exhibit additional regulation of HSC cell cycle progression. Furthermore, CD82-deficient mice exhibited increased myeloid cells and reduced B lymphocytes in the BM. The altered immune cell milieu and microenvironmental signals as a result of this distinct BM composition may additionally contribute to reduced HSC numbers and function. Myeloid-bias and a severe B lymphocyte deficit in the absence of CD82 was observed upon secondary transplant suggesting an intrinsic role for CD82 in lymphopoiesis. CD82 may also modulate important B cell survival signals, however, as CD82 associates with CD19 and major histocompatibility class I and II molecules in the plasma membrane of B lymphocytes (24,25). Thus, while CD82 may confer intrinsic differentiation and dormancy programs to HSCs, CD82 may also impact HSC function via its role in differentiated progeny and other non-hematopoietic cells, questions that will require lineage-specific knockouts to answer.
A role for DARC in HSC dormancy
CD82 activation of PKCα and ultimately TGFβ expression begged the question as to what protein may act as a ligand. Although tetraspanins are thought to primarily operate through lateral interaction within membrane domains, they do possess extracellular loops that potentially interact with neighboring cells. Prior evidence that CD82 can suppress metastases via interaction with DARC on endothelium prompted analysis of DARC in the BM. In contrast to humans, expression of DARC was not obvious in murine endothelia as assessed by flow cytometry; however, it was identified on murine BM MΦs and erythrocytes. Moreover, monocytes and MΦs were the prominent DARC+ population in human cord blood. DARC on MΦs was shown to interact with CD82 both in vitro and through imaging analysis of murine BM where DARC+ MΦs were found in contact with CD82+ HSCs. To test the roles of CD82 and DARC in controlling HSC dormancy in response to stress, a single injection of 5-fluoruracil was administered. A corresponding decrease in both CD82+ HSCs and DARC+ MΦs was observed, culminating in HSC proliferation during recovery. Coordinated return of both CD82+ LT-HSCs and DARC+ MΦs, and their close proximity, suggested an instructional relationship. The authors provide evidence that DARC maintains CD82 expression on HSCs by preventing ubiquitination and degradation of CD82. In addition, recombinant human DARC (rhDARC) protein was able to maintain CD82 expression and confer protection against cell cycle entry and proliferation. Intriguingly, complete loss of CD82 could not be rescued by rhDARC, but low levels of CD82 could be increased by addition of rhDARC. This finding suggests that CD82 present in the plasma membrane is physically stabilized by rhDARC and may serve as a dock for recycling CD82. The ability of rhDARC to preserve dormancy offers a novel therapeutic strategy for exogenous treatment of HSCs to improve HSC transplantation.
DARC is a so-called silent chemokine receptor able to bind chemokines, but not signal, thereby by acting as a sink for chemokines (26). Despite normal hematological parameters and tissue histology, mice lacking DARC are more sensitive to endotoxemia, likely owing to enhanced granulocytic infiltrates (27) and increased local concentrations of chemokines (28). DARC also sequesters chemokines for subsequent release, and evidence in humans revealed a role for DARC in regulating the circulating levels of monocyte chemoattractant protein (MCP-1) (29). It will be important to clarify any additional role for DARC in regulating HSC function in vivo through its ability to control the inflammatory state of the BM microenvironment.
MΦs—new members of the HSC niche club
MΦs are emerging as important regulators of HSC function and location in adult bone marrow (5,30-33). To specifically test whether DARC+ MΦ depletion regulates CD82 expression, the authors administered Clodronate-loaded liposomes (Clod-lip). Clod-lip-mediated MΦdepletion was previously shown to increase HSPCs in circulation (30,31) and more recently to expand the pool of HSCs in the BM (33). Consistent with the observation that loss of CD82 initiates entry to the cell cycle and increased proliferation, McCabe et al. observed increased HSC proliferation 24 h post-Clod-lip administration, and, interestingly, a loss of α4β1 expression (33). The inflammatory signal G-CSF, well-known for its mobilizing properties, also reduces MΦs in the BM and increases HSC proliferation expanding the HSC pool in the BM (33,34). Therefore, the observation that MΦ depletion resulted in a striking loss of HSCs in the BM was somewhat surprising (3). One possible explanation for the difference in BM HSC numbers upon MΦ depletion between these two studies may be due to distinct routes of administration of Clod-lip. Prior publications delivered Clod-lip intravenously, whereas Hur et al. used the intraperitoneal route. Injection via the peritoneal cavity may elicit inflammation at the site of injection, creating a demand for myeloid cells and increased differentiation of HSPCs. Increased proliferation upon loss of contact with DARC in combination with increased differentiation may result in a loss of HSCs, accounting for the observations made by Hur et al. In addition, inflammatory cues act in concert to mobilize HSCs and HSPCs as was shown by the combined effect of Clod-lip-mediated MΦ depletion and G-CSF (31). The impact of the DARC-CD82 axis on mobilization of HSPCs was not reported and it is possible that the loss of CD82 expression on HSCs due to removal of DARC+ MΦs results in enhanced circulating HSPCs.
A subset of MΦs have previously been linked with quiescent HSCs via expression of cyclooxygenase 2 (COX-2) (35), and DARC+ MΦs appear to express the highest COX-2 demonstrating functional overlap with α-SMA+ macrophages. This raises the question as to whether DARC+ MΦs exert their effects on HSC via mechanisms in addition to CD82 ligation. It is also important to note that the MΦs interrogated by Hur et al. contain only a portion of resident MΦs, as a significant number of MΦs exist in the CD11blo/neg fraction of BM (33,36). Thus, it will be important to validate the in vitro findings using lineage-specific DARC-deficient mice to test the specific role(s) of MΦs and other cell types, including erythrocytes, in maintaining HSC dormancy in vivo. The possibility of targeting MΦs for therapeutic manipulation of HSC function is exciting and warrants continued investigation.
Summary
The findings by Hur et al. add valuable insight to an emerging paradigm that MΦs regulate HSC dormancy and function (Figure 1). A number of intriguing questions remain about the CD82-DARC axis. How does the interaction between DARC and CD82 impact the bone marrow microenvironment? A dysfunctional bone marrow microenvironment can initiate myelodysplastic disease and leukemia [reviewed in (37,38)] raising important questions regarding the role(s) of MΦs in pathogenesis of leukemia. What regulates DARC expression on BM MΦs? Interferon gamma (IFNγ) maintains MΦ numbers in the BM during inflammation, but results in a loss of HSCs via enhanced proliferation (33). It is interesting to speculate that IFNγ may negatively regulate DARC expression, ultimately releasing HSCs from their quiescent state. Alternatively, IFNγ and other factors may modulate DARC-mediated chemokine binding, thus influencing the local inflammatory state. The answers to these and other questions may help facilitate therapeutic targeting of the CD82-DARC axis for improving HSC transplantation, eliminating leukemic stem cells, and manipulating HSC function in vivo.
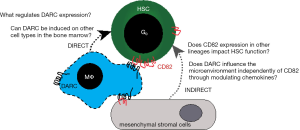
Acknowledgements
Many thanks to the very helpful discussions with Julianne N.P. Smith and Amanda McCabe.
Funding: This work was supported by National Institutes of Health R01 GM105949 to KC MacNamara.
Footnote
Conflicts of Interest: The author has no conflicts of interest to declare.
References
- Simsek T, Kocabas F, Zheng J, et al. The distinct metabolic profile of hematopoietic stem cells reflects their location in a hypoxic niche. Cell Stem Cell 2010;7:380-90. [Crossref] [PubMed]
- Kim B, Boo K, Lee JS, et al. Identification of the KAI1 metastasis suppressor gene as a hypoxia target gene. Biochem Biophys Res Commun 2010;393:179-84. [Crossref] [PubMed]
- Hur J, Choi JI, Lee H, et al. CD82/KAI1 maintains the dormancy of long-term hematopoietic stem cells through interaction with DARC-expressing macrophages. Cell Stem Cell 2016;18:508-21. [Crossref] [PubMed]
- Takubo K, Goda N, Yamada W, et al. Regulation of the HIF-1alpha level is essential for hematopoietic stem cells. Cell Stem Cell 2010;7:391-402. [Crossref] [PubMed]
- McCabe A, MacNamara KC. Macrophages: key regulators of steady-state and demand-adapted hematopoiesis. Exp Hematol 2016;44:213-22. [Crossref] [PubMed]
- Charrin S, Jouannet S, Boucheix C, et al. Tetraspanins at a glance. J Cell Sci 2014;127:3641-8. [Crossref] [PubMed]
- Bandyopadhyay S, Zhan R, Chaudhuri A, et al. Interaction of KAI1 on tumor cells with DARC on vascular endothelium leads to metastasis suppression. Nat Med 2006;12:933-8. [Crossref] [PubMed]
- Tsai YC, Mendoza A, Mariano JM, et al. The ubiquitin ligase gp78 promotes sarcoma metastasis by targeting KAI1 for degradation. Nat Med 2007;13:1504-9. [Crossref] [PubMed]
- Yang X, Wei LL, Tang C, et al. Overexpression of KAI1 suppresses in vitro invasiveness and in vivo metastasis in breast cancer cells. Cancer Res 2001;61:5284-8. [PubMed]
- Risinger JI, Custer M, Feigenbaum L, et al. Normal viability of Kai1/Cd82 deficient mice. Mol Carcinog 2014;53:610-24. [PubMed]
- Wang XQ, Yan Q, Sun P, et al. Suppression of epidermal growth factor receptor signaling by protein kinase C-alpha activation requires CD82, caveolin-1, and ganglioside. Cancer Res 2007;67:9986-95. [Crossref] [PubMed]
- Lin KK, Rossi L, Boles NC, et al. CD81 is essential for the re-entry of hematopoietic stem cells to quiescence following stress-induced proliferation via deactivation of the Akt pathway. PLoS Biol 2011;9:e1001148. [Crossref] [PubMed]
- Larochelle A, Gillette JM, Desmond R, et al. Bone marrow homing and engraftment of human hematopoietic stem and progenitor cells is mediated by a polarized membrane domain. Blood 2012;119:1848-55. [Crossref] [PubMed]
- Termini CM, Cotter ML, Marjon KD, et al. The membrane scaffold CD82 regulates cell adhesion by altering α4 integrin stability and molecular density. Mol Biol Cell 2014;25:1560-73. [Crossref] [PubMed]
- Grassinger J, Haylock DN, Storan MJ, et al. Thrombin-cleaved osteopontin regulates hemopoietic stem and progenitor cell functions through interactions with alpha9beta1 and alpha4beta1 integrins. Blood 2009;114:49-59. [Crossref] [PubMed]
- Papayannopoulou T, Priestley GV, Nakamoto B. Anti-VLA4/VCAM-1-induced mobilization requires cooperative signaling through the kit/mkit ligand pathway. Blood 1998;91:2231-9. [PubMed]
- Potocnik AJ, Brakebusch C, Fässler R. Fetal and adult hematopoietic stem cells require beta1 integrin function for colonizing fetal liver, spleen, and bone marrow. Immunity 2000;12:653-63. [Crossref] [PubMed]
- Arroyo AG, Yang JT, Rayburn H, et al. Alpha4 integrins regulate the proliferation/differentiation balance of multilineage hematopoietic progenitors in vivo. Immunity 1999;11:555-66. [Crossref] [PubMed]
- Yang YG, Sari IN, Zia MF, et al. Tetraspanins: spanning from solid tumors to hematologic malignancies. Exp Hematol 2016;44:322-8. [Crossref] [PubMed]
- Nishioka C, Ikezoe T, Furihata M, et al. CD34+/CD38− acute myelogenous leukemia cells aberrantly express CD82 which regulates adhesion and survival of leukemia stem cells. Int J Cancer 2013;132:2006-19. [Crossref] [PubMed]
- Marjon KD, Termini CM, Karlen KL, et al. Tetraspanin CD82 regulates bone marrow homing of acute myeloid leukemia by modulating the molecular organization of N-cadherin. Oncogene 2016;35:4132-40. [Crossref] [PubMed]
- Nishioka C, Ikezoe T, Yang J, et al. CD82 regulates STAT5/IL-10 and supports survival of acute myelogenous leukemia cells. Int J Cancer 2014;134:55-64. [Crossref] [PubMed]
- Nishioka C, Ikezoe T, Takeuchi A, et al. The novel function of CD82 and its impact on BCL2L12 via AKT/STAT5 signal pathway in acute myelogenous leukemia cells. Leukemia 2015;29:2296-306. [Crossref] [PubMed]
- Horváth G, Serru V, Clay D, et al. CD19 is linked to the integrin-associated tetraspans CD9, CD81, and CD82. J Biol Chem 1998;273:30537-43. [Crossref] [PubMed]
- Szöllósi J, Horejsí V, Bene L, et al. Supramolecular complexes of MHC class I, MHC class II, CD20, and tetraspan molecules (CD53, CD81, and CD82) at the surface of a B cell line JY. J Immunol 1996;157:2939-46. [PubMed]
- Novitzky-Basso I, Rot A. Duffy antigen receptor for chemokines and its involvement in patterning and control of inflammatory chemokines. Front Immunol 2012;3:266. [Crossref] [PubMed]
- Dawson TC, Lentsch AB, Wang Z, et al. Exaggerated response to endotoxin in mice lacking the Duffy antigen/receptor for chemokines (DARC). Blood 2000;96:1681-4. [PubMed]
- Lee JS, Wurfel MM, Matute-Bello G, et al. The Duffy antigen modifies systemic and local tissue chemokine responses following lipopolysaccharide stimulation. J Immunol 2006;177:8086-94. [Crossref] [PubMed]
- Schnabel RB, Baumert J, Barbalic M, et al. Duffy antigen receptor for chemokines (Darc) polymorphism regulates circulating concentrations of monocyte chemoattractant protein-1 and other inflammatory mediators. Blood 2010;115:5289-99. [Crossref] [PubMed]
- Winkler IG, Sims NA, Pettit AR, et al. Bone marrow macrophages maintain hematopoietic stem cell (HSC) niches and their depletion mobilizes HSCs. Blood 2010;116:4815-28. [Crossref] [PubMed]
- Chow A, Lucas D, Hidalgo A, et al. Bone marrow CD169+ macrophages promote the retention of hematopoietic stem and progenitor cells in the mesenchymal stem cell niche. J Exp Med 2011;208:261-71. [Crossref] [PubMed]
- Christopher MJ, Rao M, Liu F, et al. Expression of the G-CSF receptor in monocytic cells is sufficient to mediate hematopoietic progenitor mobilization by G-CSF in mice. J Exp Med 2011;208:251-60. [Crossref] [PubMed]
- McCabe A, Zhang Y, Thai V, et al. Macrophage-lineage cells negatively regulate the hematopoietic stem cell pool in response to interferon gamma at steady state and during infection. Stem Cells 2015;33:2294-305. [Crossref] [PubMed]
- Winkler IG, Pettit AR, Raggatt LJ, et al. Hematopoietic stem cell mobilizing agents G-CSF, cyclophosphamide or AMD3100 have distinct mechanisms of action on bone marrow HSC niches and bone formation. Leukemia 2012;26:1594-601. [Crossref] [PubMed]
- Ludin A, Itkin T, Gur-Cohen S, et al. Monocytes-macrophages that express α-smooth muscle actin preserve primitive hematopoietic cells in the bone marrow. Nat Immunol 2012;13:1072-82. [Crossref] [PubMed]
- Crocker PR, Gordon S. Isolation and characterization of resident stromal macrophages and hematopoietic cell clusters from mouse bone marrow. J Exp Med 1985;162:993-1014. [Crossref] [PubMed]
- Balderman SR, Calvi LM. Biology of BM failure syndromes: role of microenvironment and niches. Hematology Am Soc Hematol Educ Program 2014;2014:71-6.
- Calvi LM, Link DC. The hematopoietic stem cell niche in homeostasis and disease. Blood 2015;126:2443-51. [Crossref] [PubMed]
Cite this article as: MacNamara KC. Shedding light on HSC dormancy—a role for the DARC. Stem Cell Investig 2016;3:40.