Femur bone marrow from brain death deceased donors as source of human mesenchymal stromal cells for cell therapy
Introduction
Mesenchymal stromal cells (MSC) constitute a heterogeneous population of adult multipotent cells that were first isolated from bone marrow (BM) by Friedenstein in 1968 and described as adherent fibroblast-like cells with the ability to form colonies (colony-forming fibroblastic unit; CFU-F) and bone (1,2). Later, Haynesworth et al. described MSC in human BM and further research demonstrated their potential to differentiate into mesodermal lineages, such as osteoblasts, chondrocytes and adipocytes (3). Subsequent investigations established that human MSC (hMSC) can be isolated from several tissues. In fact, hMSC are believed to be located in the connective tissue of most organs and reside in small numbers among other cells (4).
At present, there are over 1,454 MSCs or mesenchymal stem cell interventional studies listed according to the US National Institute of Health–Clinical Trials database (Home—ClinicalTrials.gov). hMSC cells only exist in limited quantities in situ, therefore, in order to obtain therapeutically effective cell doses, hMSC are typically manipulated by in vitro cell expansion. BM obtained from the iliac crests (ICBM) has been one of the most frequent sources for isolation and expansion of hMSC. This anatomical site was the first and most widely used in BM harvesting, being accepted as the “gold standard”. BM heterogeneity in humans has been well documented. A study by Veyrat-Masson et al. compared the proportion of clonogenic progenitors (CFU-F) of ICBM in relation to femoral head spongy bone collected during hip arthroplasty and showed that the latter contained a higher amount of CFU-F (5).
Allogeneic hMSC (allo-hMSC) offer practical advantages over autologous cells for cell therapy since the administration of allo-hMSC would avoid the inconvenience of cell collection procedures to the patient and expansion timeout. In addition, the tissue source can be previously selected and validated to maximize potency and safety, making it a readily available product for treatment in acute-phase disease (6). Thus, the amount of hMSC from adult tissues may be limited when obtained from a living donor and therefore the supply is also very limited (7,8). Research on an alternative source of hMSC instead of living donors, such as a deceased donor (DD), has not been fully explored and could be relevant (9).
The brain-dead organ donor is a distinct and challenging pathophysiologic condition that can affect organs, tissues and cells. Difficulties in managing the failure of multiple organ donors is one of the main reasons for poor yield in organ and tissue procurement. Brain death (BD) is a pathological process which is characterized by hormonal impairment, hemodynamic imbalance, and a systemic inflammatory response (10). Obtaining organs and tissues for transplantation from BD-DD is a widely accepted strategy; however, during the routine BD-DD process, procuring the BM is not performed. Therefore, the use of BM from BD-DD as an alternative source of allo-hMSC is promising but has been little explored (11-15). The aim of the present study was to evaluate the potential of femur BM (FBM) from BD-DD as a source of hMSC for cell therapy. To our knowledge, there are no previous studies evaluating BM from femur DD as a source of hMSC. We analyzed the relative potential of the starting material, phenotypic profile, proliferation and differentiation capacity of hMSC obtained from matched FBM and ICBM from BD-DD. We present this article in accordance with the STROBE reporting checklist (available at https://sci.amegroups.com/article/view/10.21037/sci-2023-003/rc).
Methods
Subjects and BM collection
All the procedures were done in accordance with the Declaration of Helsinki (as revised in 2013) and the Uruguay national standards for donation and transplantation. This project is legally framed in the donation and transplantation laws of Uruguay (Law Nos. 14005/1971, 17668/2003, 18362/2008, and 18968/2012) and regulatory decrees on safety and traceability of tissues and cells (Decree No. 160/2006). Confirmation of deaths were carried out by authorized technicians. Article 1 and 4 of Uruguayan law No. 18968/2012 establish that explicit consent was not required for DDs. They give their presumed consent for the donation of cells, organs and tissues for therapeutic or scientific purposes. The study was approved by the National Ethic Committee from the Instituto Nacional de Donación y Trasplante (INDT, Ministry of Health, Uruguay, V3-22032018, https://www.indt.gub.uy).
BD donors selected for organ and tissue donation according to the INDT criteria (Table S1) were included provided that they met additional inclusion criteria for this study, age between 18–70 years and less than 24 h from death for processing. Donor-matched ICBM and FBM were collected from eight DDs.
Samples of BM were harvested at the surgical room during standard tissue ablation procedures. ICBM samples were collected by curettage of the anterior iliac crests. After asepsis of the incisional zone, fragments of spongy bone were obtained using a curette soaked in saline solution with 100 IU/mL of heparin and transferred immediately into conical tubes containing 20 mL of alpha-MEM GlutaMax (Gibco, USA) with 800 IU/mL of heparin (Fu, Uruguay). FBM samples were obtained by curettage of the proximal femur epiphysis using a similar procedure. Conical tubes containing samples were manually shaken to promote further release of BM cells into the media, then placed at 4 °C in an isothermic box and transported to the laboratory.
BM processing
Samples were processed under aseptic conditions in a biological safety cabinet. The tubes containing samples were weighed and sample size was calculated by subtracting the weight of the empty collection tubes. Collected BM samples were diluted a minimum of 1:5 with alpha-MEM GlutaMax supplemented with 1% penicillin/streptomycin (Gibco, USA) and mixed, inverting the tube several times. Cell suspensions were then filtered through a 70 µm cell strainer (Corning, USA) to remove bone splinters.
Mononuclear cells (MNC) were isolated from each BM sample using Ficoll-Paque Premium (GE, Sweden, density =1.077 g/mL) by the density gradient method. Briefly, filtered cell suspension was layered on Ficoll-Paque and centrifuged at 400 g for 40 min without break at room temperature. The MNC layer was collected by a sterile pipette, washed with Dulbecco’s phosphate-buffered saline (DPBS; Gibco) and centrifuged two times at 100 g for 10 min. Finally, MNC were re-suspended in supplemented culture media. The amount of nucleated cells (NC) was counted on every sample by both manual and automatic procedures. Following red cell lysis in 3% acetic acid with methylene blue, a manual count of NC was performed using a Neubauer chamber. Automatic leukocyte count was performed using an automatic cell counter (Cell-dyn Hematology Analyzer, Abbott). NC counts were calculated by averaging the results of both methods.
CFU-F assay
For CFU-F assay, isolated NC were resuspended in alpha-MEM supplemented with 15% fetal bovine serum (FBS) (Invitrogen, Brazil) at concentrations of 2.5×105 and 5×105 cells/mL; 2 mL of each cell suspension was plated in triplicate into 35 mm diameter petri dishes. Cells were cultured at 37 °C in a humidified atmosphere with 5% CO2 and media was changed after seven days. On the 14th day cells were washed with phosphate-buffered saline (PBS), fixed with methanol and stained with 1% crystal violet diluted in methanol. Colonies of more than 50 cells were manually counted using a magnifying glass.
hMSC expansion
For in vitro hMSC cultures, isolated NC were seeded into tissue culture flasks (Nunc EasYFlask, Thermo Fisher Scientific, Denmark) at a density of 2–4×105 cells/cm2 using alpha-MEM GlutaMax supplemented with 15% FBS (Invitrogen) and 1% Penicillin/Streptomycin. Cell cultures were maintained at 37 °C and 5% CO2. After 1 week, the supernatant containing non-adherent cells was removed, cells were rinsed with DPBS and fresh complete medium was added. Cells were expanded until 80% confluency, rinsed with DPBS and harvested using TrypleTM Select (Gibco, Denmark). For further passages, cells were manually counted and seeded at a density of 4,000 hMSC/cm2 and grown in a medium containing 15% FBS. hMSC total yield was calculated per gram of processed tissue at passage 0 (P0), passage 1 (P1) and passage 2 (P2).
Phenotypic analysis of hMSC by flow cytometry
hMSC from passages 2 and 3 were dissociated using TrypLETM Select and suspended at 1×107 cells/mL in PBS-ethylenediaminetetraacetic acid (EDTA). Immunophenotypic analysis was performed using Stemflow hMSC Analysis Kit (BD, catalog #562245, USA) following manufacturer instructions, see Table S2. Briefly, 100 µL of cell suspension was incubated for 30 min at room temperature with a mix of antibodies positive for hMSC (CD90 FITC, CD105 PerCP-Cy5.5, CD73 APC), a negative cocktail of antibodies (CD34 PE/CD11b PE/CD19 PE/CD45 PE/HLA-DR PE), as well as isotype positive and negative control cocktails. After incubation, cells were washed with PBS-EDTA supplemented with 1% FBS and 0.09% of sodium azide, centrifuged and suspended in PBS for flow cytometry analysis. Samples were acquired using a FACSCalibur flow cytometer (BD, USA) and data analyzed with CellQuest Pro software (BD, USA).
Analysis of HLA expression
A routine serological complement-dependent cytotoxicity (CDC) typing method with allele-specific monoclonal antibody and complement were able to investigated allele-specific expression of HLA-A or -B at protein levels on hMSC tested by their massive killing with relevant anti-HLA-A or B antibody. Briefly, hMSC from passages 2 and 3 were dissociated using TrypLETM Select and resuspended at 2–3×103 cells/µL in Veronal buffer; 1 µL of cell suspension was added to each well of the Terasaki HLA Tissue Typing Trays, Lambda monoclonal typing Tray set class I (catalog # LM144A and LM144B) and class II (catalog # MDR172) (One Lambda Inc., USA). After 1 hour of incubation at room temperature, 5 µL of Fluoro Quench (One Lambda Inc., catalog# FQAE500, USA) was added. The readout was performed by fluorescence microscopy.
Differentiation assays
Differentiation of hMSC into the adipogenic, chondrogenic, and osteogenic lineages were performed as Russell et al. described with some modifications (16).
Briefly, for osteogenic differentiation, hMSC from passages 2 and 3 were seeded in duplicate at a density of 4.2×103 hMSC/cm2 and expanded until 50–75% confluency was reached. Osteogenesis was induced with alpha-MEM GlutaMax supplemented with 10% FBS, 1% Penicillin/Streptomycin, 100 nM dexamethasone (Sigma-Aldrich, Bioxtra, USA), 10 mM beta-glycerophosphate (Calbiochem, USA), and 50 µM L-ascorbic acid 2-phosphate (Sigma-Aldrich, Japan). The medium was changed 2 times per week. On day 21 of culture in osteogenic medium, hMSC were fixed in neutral buffered formalin 10% for 30 minutes and stained with 1% Alizarin Red S (pH 4.2, Sigma-Aldrich, China) for 10 minutes to detect calcified extracellular matrix.
For adipogenic differentiation, hMSC from passages 2 and 3 were seeded in duplicate at density of 2.1×104 hMSC/cm2, expanded until 100% confluency and differentiated over 21 days in adipogenic medium: alpha-MEM GlutaMax supplemented with 17% FBS, 1% Penicillin/Streptomycin, 0.5 µM dexamethasone, 0.5 mM isobutylmethylxanthine (Sigma BioUltra, USA), and 50 µM indomethacin (Sigma-Aldrich, USA). The medium was changed 2 times per week. To detect lipid accumulation, cultures were fixed in neutral buffered formalin 10% for 30 min and stained with 0.2% Oil Red O (Sigma-Aldrich, USA) in 60% isopropanol (Biopack, Argentina) for 10 min.
Chondrogenic differentiation was induced in pellet cultures of hMSC from passages 2 and 3. Conical tubes in duplicate with 2.5×105 cells/tube and 0.5 mL/tube of chondrogenic medium were centrifuged at 200 g for 5 min at room temperature for pellet formation. Cell pellets were induced in high glucose DMEM (Gibco, USA) supplemented with 10 ng/mL of transforming growth factor-beta 3 (Sigma, SRP3171, USA), 100 nM dexamethasone, 100 µM of L-ascorbic acid 2-phosphate, 2 mM glutamine (Gibco, USA), 1 mM of pyruvate (Sigma Life, USA), and 10 µL/mL of insulin-transferrin-sodium selenite liquid media supplement (ITS+1) (Sigma, I2521, USA). Medium was changed 3 times per week. Three weeks after culture in differentiation medium, pellet cultures were fixed in 10% buffered formalin, embedded in paraffin, sectioned at 5 µm, deparaffinized, and hydrated before staining. Sulfated proteoglycan deposition (purple metachromasia) in the extracellular matrix was assessed by 1% toluidine blue (Sigma-Aldrich, India) staining. Qualitative analysis was performed by microscopy using Nikon Tis. Adipogenic and osteogenic differentiation was performed from iliac crest (n=6) and femur (n=4) and for chondrogenic differentiation from iliac crest (n=7) and femur (n=5). Parent hMSC was maintained in growth medium supplemented with serum without differentiation factors as negative controls.
Statistical analysis
Data were analyzed using descriptive statistics. Results were expressed as means ± standard deviations, percentages, and ranges. Comparisons between paired variables of donor-matched ICBM and FBM groups were tested using Wilcoxon signed ranks test or paired t-test where variables had a normal distribution. P<0.05 was considered statistically significant. Plots and statistical analysis were performed using GraphPad Prism 8.
Results
Analysis of starting material and hMSC yields from FBM and ICBM samples
Sixteen donor-matched ICBM and FBM samples were processed from eight DDs as described in Table 1. An average of 3.88×107±3.46×107 NC per gram and 1.46×107±1.03×107 NC per gram were obtained in ICBM and in FBM samples, respectively (Figure 1A). These freshly isolated NC presented clonal proliferative potential as determined by CFU-F assay. The average CFU-F frequency was 0.0057%±0.0042% in ICBM NC and 0.0042%±0.0036% in FBM NC (Figure 1B). Neither the amount of NC per gram (P≥0.09) nor the frequency of CFU-F differ significantly (P≥0.73) in freshly isolated cells from ICBM or FBM. These findings demonstrate the ability to obtain viable and proliferative hMSC isolated from DDs from both sources.
Table 1
Donor ID/statistical data | Donor age (years) |
Gender+ (% male: 37.5%) | Cause of death | PMI$ | Iliac crest BM weight (grams) | Femur BM weight (grams) | Total CFU-F per iliac crest processed sample | Total CFU-F per femur processed sample |
---|---|---|---|---|---|---|---|---|
D4 | 20 | F | Polytrauma, traffic accident | 1,080 | 3.92 | 1.24 | 5,329 | 1,064 |
D5 | 59 | M | Non-traumatic subdural hemorrhage | 510 | 1.93 | 1.34 | 7,107 | 97 |
D6 | 67 | M | Spontaneous intracranial hemorrhage | 330 | 0.32 | N.D. | N.D. | N.D. |
D7 | 18 | F | Acute asthma | 960 | 0.6 | 0.82 | 252 | 77 |
D8 | 45 | F | Non-traumatic intracerebral hemorrhage | 940 | 1.08 | 2.26 | 208 | 1,398 |
D9 | 53 | M | Roof fall | 1,280 | 1.71 | 1.72 | 5,061 | 806 |
D10 | 44 | F | Intracerebral hemorrhage, basal ganglia hemorrhage | 1,300 | 0.35 | 0.15 | 1,357 | N.D. |
D11 | 42 | F | Subarachnoid hemorrhage | 1,110 | 2.1 | 2.86 | 105 | 322 |
Mean | 44 | 939 | 1.50 | 1.48 | 2,774 | 627 | ||
SD | 17 | 349 | 1.20 | 0.90 | 2,961 | 547 | ||
Median | 45 | 1,020 | 1.40 | 1.34 | 1,357 | 564 | ||
Range | 18–67 | 330–1,300 | 0.32–3.9 | 0.15–2.89 | 105–7,107 | 77–1,398 |
In this graph the statistical analysis was unpaired because some values were N.D. *, this statistical analysis was unpaired; +, M: male, F: female; $, PMI: is defined as the time since death to the start of BM processing in minutes; PMI, postmortem interval; BM, bone marrow; CFU-F, colony-forming fibroblastic unit; D, donor; N.D., not determined; SD, standard deviation.
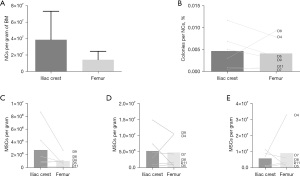
Either ICBM or FBM hMSC from DD successfully grew in culture. hMSC from DD were expanded, considering initial plating as P0. The comparative analysis of hMSC yields showed that there were no significant differences in hMSC obtained per gram of BM when comparing IC with F samples, in P0 (P=0.17), P1 (P=0.82) and P2 (P>0.99) (Figure 1C-1E). At P2, 12.5×106±12.9×106 hMSC per gram of BM were obtained from FBM after 22.6±8.0 days and 5.0×106±4.4×106 hMSC per gram of BM were obtained from ICBM after 21.1±9.2 days.
Phenotypic characterization of hMSC generated from ICBM and FBM
Spindle-shaped cells with the ability to adhere to plastic were observed after in vitro culture of MNC isolated from FBM and ICBM samples of DD (Figure 2). Cultured cells derived from both sources expressed C90, CD73, CD105, but not CD45, CD34, CD11b, CD19 and HLA-DR markers by flow cytometry analysis (Figure 3). There were no statistically significant differences in the percentage of positive cells when comparing hMSC obtained from FBM vs. ICBM for all the markers analyzed. Additionally, the cell-surface expression of HLA class I and II tested by complement dependent cytotoxicity (CDC) assay was evaluated. HLA class I and II expression on lymphocytes derived from the same donor was analyzed as well. HLA-A antigens expression on hMSC was clearly defined and comparable with that of donor lymphocytes. However, HLA-B antigens were very weakly expressed or undetectable on hMSC samples. Expression of HLA-DR antigens were not detectable on hMSC (Table 2).
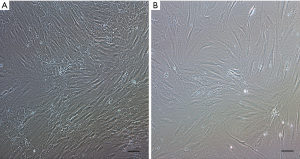
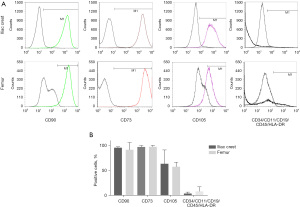
Table 2
Donor ID | Source | Cell type | Cell-surface expression of HLA class I | Cell-surface expression of HLA class II | ||
---|---|---|---|---|---|---|
HLA-A serotype | HLA-B serotype | HLA-DR serotype | ||||
D4 | Iliac crest BM | hMSC | A2, A29(19) | Bw6 | ND | |
Peripheral blood | Lymphocyte | A2, A29(19) | B44(12)-Bw4, B60(40)-Bw6 | DR7, DR15(2) | ||
D8 | Iliac crest BM | hMSC | A3, A23(9) | ND | ND | |
Femur BM | hMSC | A3, A23(9) | ND | ND | ||
Peripheral blood | Lymphocyte | A3, A23(9) | B7-Bw6 | DR7, DR15(2) | ||
D10 | Iliac crest BM | hMSC | A2, A24(9) | ND | ND | |
Femur BM | hMSC | A2, A24(9) | ND | ND | ||
Peripheral blood | Lymphocyte | A2, A24(9) | B7-BW6, B35-Bw6 | DR13(6), DR16(2) |
Analysis of the expression was performed by complement dependent cytotoxicity assay in lymphocytes from peripheral blood and hMSC from passages two or three from the same donor. HLA, human leukocyte antigen; HLA -A, HLA type A; HLA-B, HLA type B; HLA-DR, isotype of HLA clase II; hMSC, human mesenchymal stromal cells; BM, bone marrow; ND, not detected.
Differentiation assays of hMSC
The trilineage potential of hMSC obtained from FBM and ICBM was evaluated using the differentiation conditions specified above. Representative images of the results obtained are shown in Figure 4. All tested samples derived from FBM or ICBM sources induced adipogenic differentiation. Red lipid drops were detected using Oil Red O as shown in Figure 4A,4D. In controls without an induction medium, lipid drops were not observed (Figure 4A1 and Figure 4D1). For osteogenic differentiation analysis, calcification of the extracellular matrix was observed when staining with Alizarin Red D after induction of hMSC derived from both sources (Figure 4B,4E). Otherwise, hMSC negative controls remained unchanged (Figure 4B1 and Figure 4E1). Metachromasia (strong pink) produced by the presence of proteoglycans due to chondrogenic differentiation was observed in both sources when the induced hMSC pellets were stained with toluidine blue (Figure 4C,4F). Proteoglycans were not detected in controls without induction (blue color), as shown in Figure 4C1 and Figure 4F1. In view of these results, DD-hMSC obtained from both sites, FBM or ICBM, showed trilineage differentiation potential, without qualitative differences between them.
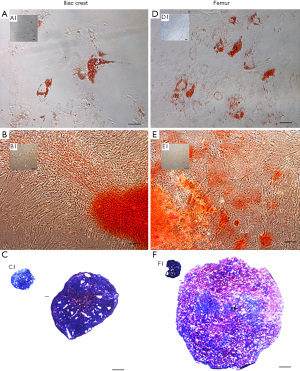
Discussion
FBM and ICBM from BD-DD as an alternative source of hMSC for cell therapy was evaluated. The results confirmed that it is possible to isolate and expand cells from FBM that comply with the in vitro characteristics of hMSC.
No significant differences were observed in the amount of NC obtained per gram of BM when comparing both sources, even though there was a tendency to be lower in FBM. Values of NC obtained per gram of BM were in the same range as those reported in other studies of deceased or live donors (17,18). In addition, the average content of mesenchymal progenitors quantified by CFU-F assay from DD (0.0057%±0.0042% in ICBM and 0.0042%±0.0036% in FBM) was comparable to the ratio of hMSC in relation to NC reported for live donors (0.001–0.01%) (19). Furthermore, no significant differences in the content of mesenchymal progenitors attributed to the source (ICBM vs. FBM) were observed. In contrast to these results, Veyrat-Masson et al. reported a higher number of CFU-F in FBM from live donors compared to ICBM (5). This discrepancy could be attributed to differences in the harvesting methods applied and the lack of sample matching of the mentioned study. Vasiliadis et al. noted that direct comparison of isolated cell numbers between different studies may be difficult, as differences in isolation methods, culture times, and culture media used, led to variable results (18). However, yields hMSC attained in this study are comparable to those reported with BM collected from living donors from FBM (20).
DD-derived hMSCs expressed HLA class I molecules and did not express HLA class II molecules, as reported for non-stimulated hMSC from living donors (21). This result differs from the findings of Cavallo et al., who reported that hMSC from dead donors did not express HLA-ABC, as judged by staining with the HLA-ABC antibody (11). HLA-A expression in hMSC using the CDC assay, was detected while HLA-B expression was very low or undetectable, as reported in other studies in living donors (22,23). This result contrasts with expression of HLA-A and -B found on lymphocytes. Isa et al. in 2010 suggested that lack of constitutive HLA-B expression on hMSC could be influencing the induction of peripheral tolerance towards HLA-B restricted peptides. They propose that a major contributor to repression of HLA-B in stem cells during basal non-induced conditions may be a post-transcriptional mechanism leading to translational attenuation in stem cells. Since different HLA alleles have variable affinities for intracellularly-generated peptides, the lack of HLA-B expression can influence antigen presentation and the resulting immune response (22).
The cells obtained from FBM were able to differentiate in vitro into adipocytes, chondroblasts, and osteoblasts and presented a phenotype in accordance with the criteria proposed by the International Society for Cell & Gene Therapy (24). These results are consistent with other studies that characterized hMSC from ICBM DDs (11). Moreover, novel data which arise from the analysis of this study are that cells obtained from FBM from DD are phenotypically and functionally equivalent to hMSC obtained from ICBM. These findings support the use of FBM as a source of hMSC, as well as ICBM. The fact that FBM from DDs is a suitable source for producing hMSC may have a promising potential clinical utility.
Small sample size is a limitation of this study, but we applied the strategy of using donor-matched FBM and ICBM samples for hMSC isolation to avoid reported donor-to-donor variability. On the other hand, to minimize weight differences between samples, we could have removed trabecular bone and fat fragments obtained before weighing. Alternatively, the solid fragments could have been separated by filtration and then enzymatically digested to extract any hMSC from solid fragments (25). However, the methodology with minimal manipulation used in this study was based on a clinical translational strategy. The opportunity to use allo-hMSCs from FBM DD for cell banking is of great importance. This source could allow collection of hMSC suitable for clinical application. The feasibility of isolation and expansion of FBM MSC in large-scale production for clinical application will be studied in future research. Additionally, upcoming research will investigate phenotypic safety markers such as TF/CD142 (26) and more accessible sources of hMSD in DD, and compare the results with hMSC from living donors.
Acknowledgments
The authors would like to thank Jorge Zunino from INDT for establishing the sample collection procedure; Milka Bengochea, Inés Álvarez and Mariana Lorenzo from INDT for revision of the initial project and support in CDC assays; Silvana Podestá’s team from Anatomía Patológica for assistance with histological processing and Anna Barindelli from Laboratorio Clínico for helping us with the multi-parameter automated hematology analyzer.
Funding: This study was supported by Comisión Sectorial de Investigación Científica (CSIC) (Research Grant # 94), Programa de Investigación Biomédica (PRO.IN.BIO) and Comisión Académica de Posgrado (CAP) from Universidad de la República, Uruguay.
Footnote
Reporting Checklist: The authors have completed the STROBE reporting checklist. Available at https://sci.amegroups.com/article/view/10.21037/sci-2023-003/rc
Conflicts of Interest: All authors have completed the ICMJE uniform disclosure form (available at https://sci.amegroups.com/article/view/10.21037/sci-2023-003/coif). The authors have no conflicts of interest to declare.
Ethical Statement: The authors are accountable for all aspects of the work in ensuring that questions related to the accuracy or integrity of any part of the work are appropriately investigated and resolved. All the procedures were done in accordance with the Declaration of Helsinki (as revised in 2013) and the Uruguay national standards for donation and transplantation. This project is legally framed in the donation and transplantation laws of Uruguay (Law Nos. 14005/1971, 17668/2003, 18362/2008, and 18968/2012) and regulatory decrees on safety and traceability of tissues and cells (Decree No. 160/2006). Confirmation of deaths were carried out by authorized technicians. Article 1 and 4 of Uruguayan law No. 18968/2012 establish that explicit consent was not required for deceased donors. They give their presumed consent for the donation of cells, organs and tissues for therapeutic or scientific purposes. The study was approved by the National Ethic Committee from the Instituto Nacional de Donación y Trasplante (INDT, Ministry of Health, Uruguay, V3-22032018, https://www.indt.gub.uy).
Open Access Statement: This is an Open Access article distributed in accordance with the Creative Commons Attribution-NonCommercial-NoDerivs 4.0 International License (CC BY-NC-ND 4.0), which permits the non-commercial replication and distribution of the article with the strict proviso that no changes or edits are made and the original work is properly cited (including links to both the formal publication through the relevant DOI and the license). See: https://creativecommons.org/licenses/by-nc-nd/4.0/.
References
- Friedenstein AJ, Petrakova KV, Kurolesova AI, et al. Heterotopic of bone marrow. Analysis of precursor cells for osteogenic and hematopoietic tissues. Transplantation 1968;6:230-47. [Crossref] [PubMed]
- Friedenstein AJ, Chailakhyan RK, Latsinik NV, et al. Stromal cells responsible for transferring the microenvironment of the hemopoietic tissues. Cloning in vitro and retransplantation in vivo. Transplantation 1974;17:331-40. [Crossref] [PubMed]
- Haynesworth SE, Goshima J, Goldberg VM, et al. Characterization of cells with osteogenic potential from human marrow. Bone 1992;13:81-8. [Crossref] [PubMed]
- Freedman BR, Mooney DJ. Biomaterials to Mimic and Heal Connective Tissues. Adv Mater 2019;31:e1806695. [Crossref] [PubMed]
- Veyrat-Masson R, Boiret-Dupré N, Rapatel C, et al. Mesenchymal content of fresh bone marrow: a proposed quality control method for cell therapy. Br J Haematol 2007;139:312-20. [Crossref] [PubMed]
- Newman RE, Yoo D, LeRoux MA, et al. Treatment of inflammatory diseases with mesenchymal stem cells. Inflamm Allergy Drug Targets 2009;8:110-23. [Crossref] [PubMed]
- Goodwin HS, Bicknese AR, Chien SN, et al. Multilineage differentiation activity by cells isolated from umbilical cord blood: expression of bone, fat, and neural markers. Biol Blood Marrow Transplant 2001;7:581-8. [Crossref] [PubMed]
- Miura M, Gronthos S, Zhao M, et al. SHED: stem cells from human exfoliated deciduous teeth. Proc Natl Acad Sci U S A 2003;100:5807-12. [Crossref] [PubMed]
- Mansilla E, Marín GH, Berges M, et al. Cadaveric bone marrow mesenchymal stem cells: first experience treating a patient with large severe burns. Burns Trauma 2015;3:17. [Crossref] [PubMed]
- Issac E, Venugopalan S. Pathophysiology of brain stem death. Amrita J Med 2020;16:50-4. [Crossref]
- Cavallo C, Cuomo C, Fantini S, et al. Comparison of alternative mesenchymal stem cell sources for cell banking and musculoskeletal advanced therapies. J Cell Biochem 2011;112:1418-30. [Crossref] [PubMed]
- Rao PN, Deo DD, Marchioni MA, et al. Structural and Functional Characterization of Deceased Donor Stem Cells: A Viable Alternative to Living Donor Stem Cells. Stem Cells Int 2019;2019:5841587. [Crossref] [PubMed]
- Johnstone BH, Miller HM, Beck MR, et al. Identification and characterization of a large source of primary mesenchymal stem cells tightly adhered to bone surfaces of human vertebral body marrow cavities. Cytotherapy 2020;22:617-28. [Crossref] [PubMed]
- Pereira L, Echarte L, Romero M, et al. Synthesis and characterization of a bovine collagen: GAG scaffold with Uruguayan raw material for tissue engineering. Cell Tissue Bank 2021; Epub ahead of print. [Crossref] [PubMed]
- Echarte L, Grazioli G, Pereira L, et al. Processing methods for human amniotic membrane as scaffold for tissue engineering with mesenchymal stromal human cells. Cell Tissue Bank 2022; Epub ahead of print. [Crossref] [PubMed]
- Russell KC, Phinney DG, Lacey MR, et al. In vitro high-capacity assay to quantify the clonal heterogeneity in trilineage potential of mesenchymal stem cells reveals a complex hierarchy of lineage commitment. Stem Cells 2010;28:788-98. [Crossref] [PubMed]
- Hermann PC, Huber SL, Herrler T, et al. Concentration of bone marrow total nucleated cells by a point-of-care device provides a high yield and preserves their functional activity. Cell Transplant 2008;16:1059-69. [Crossref] [PubMed]
- Vasiliadis AV, Galanis N. Human bone marrow-derived mesenchymal stem cells from different bone sources: a panorama. Stem Cell Investig 2020;7:15. [Crossref] [PubMed]
- Pittenger MF, Mackay AM, Beck SC, et al. Multilineage potential of adult human mesenchymal stem cells. Science 1999;284:143-7. [Crossref] [PubMed]
- Juneja SC, Viswanathan S, Ganguly M, et al. A Simplified Method for the Aspiration of Bone Marrow from Patients Undergoing Hip and Knee Joint Replacement for Isolating Mesenchymal Stem Cells and In Vitro Chondrogenesis. Bone Marrow Res 2016;2016:3152065. [Crossref] [PubMed]
- Klyushnenkova E, Mosca JD, Zernetkina V, et al. T cell responses to allogeneic human mesenchymal stem cells: immunogenicity, tolerance, and suppression. J Biomed Sci 2005;12:47-57. [Crossref] [PubMed]
- Isa A, Nehlin JO, Sabir HJ, et al. Impaired cell surface expression of HLA-B antigens on mesenchymal stem cells and muscle cell progenitors. PLoS One 2010;5:e10900. [Crossref] [PubMed]
- Dellgren C, Ekwelum VA, Ormhøj M, et al. Low Constitutive Cell Surface Expression of HLA-B Is Caused by a Posttranslational Mechanism Involving Glu180 and Arg239. J Immunol 2016;197:4807-16. [Crossref] [PubMed]
- Dominici M, Le Blanc K, Mueller I, et al. Minimal criteria for defining multipotent mesenchymal stromal cells. The International Society for Cellular Therapy position statement. Cytotherapy 2006;8:315-7. [Crossref] [PubMed]
- Cox G, Boxall SA, Giannoudis PV, et al. High abundance of CD271(+) multipotential stromal cells (MSCs) in intramedullary cavities of long bones. Bone 2012;50:510-7. [Crossref] [PubMed]
- Moll G, Ankrum JA, Olson SD, et al. Improved MSC Minimal Criteria to Maximize Patient Safety: A Call to Embrace Tissue Factor and Hemocompatibility Assessment of MSC Products. Stem Cells Transl Med 2022;11:2-13. [Crossref] [PubMed]
Cite this article as: Echarte L, Sujanov A, Machin D, Marquisá N, Touriño C. Femur bone marrow from brain death deceased donors as source of human mesenchymal stromal cells for cell therapy. Stem Cell Investig 2023;10:12.