Outside the brain: an inside view on transgenic animal and stem cell-based models to examine neuronal serotonin-dependent regulation of HPA axis-controlled events during development and adult stages
Serotonin (5-hydroxytryptamine, 5-HT) is one of three neurotransmitters that compose the monoaminergic neurotransmission system. Together with dopamine and norepinephrine, 5-HT modulates physiological processes that are the cornerstones of our cognitive and affective behaviour. Disturbances or even malfunctions of monoaminergic neurotransmission are involved in the pathogenesis of psychiatric diseases such as anxiety, depression, attention deficit hyperactivity disorder, schizophrenia, and addiction. The 5-HT neurotransmission system is built and maintained by a small number of morphologically heterogeneous neurons that locate to the raphé nuclei. In numbers, only roughly 20,000 serotonergic neurons in rodents and up to 500,000 serotonergic neurons in humans can be found in the raphé nuclei (1,2). Within the raphé nuclei, the serotonergic neurons are distributed over nine subpopulations localized to specific areas of the brainstem, which are labelled B1 from caudal to B9 rostrally located (3). The areas B1 to B4 comprise the serotonergic neurons of the caudal raphé nuclei. This serotonergic subpopulation has been shown to project into the brain stem and the spinal cord. The serotonergic neurons located in the areas B5 to B9 comprise the subpopulation of the dorsal and median raphé nuclei (DRN, respectively MRN). Their projections innervate nearly all brain regions, for example cortex, hippocampus and hypothalamus. A common feature of DRN and MRN serotonergic neurons is to densely innervate their projection areas with varicose axons featuring high numbers of axonal branches (4-6). The small number of serotonergic neurons in rodent brain and the extensive neurite network growth rendered this special neuronal population as not suitable for establishing primary neuronal cultures as could be easily done for example for hippocampal neurons. The generation of stem cell-derived serotonergic neurons is one experimental system to overcome this obstacle and to investigate biochemical, cellular and molecular aspects of 5-HT neurotransmission in vitro. Well established stem cell models are serotonergic neurons derived from either mouse neuronal stem cells (7,8) or from mouse embryonic stem (ES) cells (9-11), which were applied in a number of studies performed to gain insight on 5-HT neurotransmission on a cellular level. With regard to mouse neuronal stem cell-derived serotonergic neurons, these cells were successfully used in combined studies with rodent models, for example to examine the role of microRNAs in depression (12,13) or the contribution of protein kinases to the neuronal activity of DRN serotonergic neurons (14). Mouse ES cell-derived serotonergic neurons can not only be applied to perform studies on 5-HT neurotransmission in vitro (9,15). They also enable researchers to establish experimental models that are derived from transgenic animals featuring a genotype of interest, for example transgenic mice that express human wild-type or mutated serotonin transporter (16). As shown for the combination of neuronal stem cell-derived serotonergic neurons and animal models, the combination of experiments performed in transgenic mice and the corresponding genetic ES cell-derived serotonergic neurons can be applied to address research questions of interest, as done recently to further characterize the role of allosteric binding site of selective serotonin re-uptake inhibitors in the human serotonin transporter (11). Recently, a number of protocols for the generation of human fibroblast and iPS cell-derived serotonergic neurons were introduced to the scientific community [discussed and reviewed in (17,18)]. Now, with the possibility to generate human fibroblast- or induced pluripotent stem (iPS) cell-derived serotonergic neurons (19-21) a new experimental system is established that enables researchers to analyze human 5-HT neurotransmission on a molecular and cellular level. These experimental approaches provide valuable starting points for future research that will generate knowledge on how (genetically-pharmacologically defined subsets of) human serotonergic neurons function as well as on the role of genetic variants in psychiatric diseases and mechanisms of drugs applied in pharmacotherapy. Future work performed with in vitro-generated human serotonergic neurons will significantly contribute to the experimental data gained so far from transgenic animal models of serotonergic neurotransmission. Furthermore, the synergistic effects provided by the combination of stem cell-based human and rodent models for 5-HT neurotransmission will help us to unravel the unknowns of 5-HT neurotransmission and finally understand the functionality of mature serotonergic neurons in vivo, especially regarding the pathogenesis and treatment of diseases that display a defective 5-HT neurotransmission.
The effects of 5-HT signalling, or neurotransmission in general, are not restricted to the central nervous system (CNS). Just as neurons process stimuli coming from outside the CNS, neuronal activity also initiates, controls or regulates events occurring outside the CNS. North and colleagues investigated central regulatory mechanisms in hematopoietic stem (HPS) cell production during the embryonic development of zebrafish (22). Generally, the formation and maintenance of HPS cells are characterized by precisely tuned mechanisms that bring together various cellular signals and result in a balance of HPS cell self-renewal and differentiation. And although HPS cell production is tightly bound to embryonic development, and a high number of cellular and molecular interactions involved in generation and expansion of HPS cells were identified [reviewed in (23)], the helmsman of these processes during embryonic development remained in the dark. In their recent work, Kwan et al. were able to demonstrate a 5-HT-stimulated central regulation of HPS cell generation during embryonic development. Moreover, using a knockdown of brain-specific tryptophan hydroxylase 2 (TPH-2), they provided experimental data that this control depends on brain-derived 5-HT rather than 5-HT synthesized by tryptophan hydroxylase 1 (TPH-1) expressed by and functional in enterochromaffin cells. This is highly interesting since TPH-1-derived 5-HT accounts for up to 90% of 5-HT found in the body. At first glance, it would appear rather more economical if the body would rely on either a non-neuronal resource of 5-HT required for non-neuronal processes like HPS cell generation during embryonic development or a more adjacently located regulator. Fitting to their highly interesting finding of neuronal 5-HT’s involvement in HPS cell production, is the mechanism identified by Kwan and colleagues that is used by neuronal 5-HT to regulate embryonic HPS cell production: the serotonergic regulation is mediated by the stress-responsive hypothalamic-pituitary-adrenal (HPA) axis and the activity of the glucocorticoid receptor (Figure 1). Besides the sympathetic nervous system, the HPA axis is the main mediator of the body’s response to external or internal stressors. It is an autoregulative neuroendocrine system, which plays an essential role in initiation, adaptation and termination of a stress response in order to restore the body’s homeostasis (24,25). The HPA axis and the serotonergic neurotransmission system were shown to exert reciprocal regulatory mechanisms. On the side of serotonergic neurons, 5-HT signaling modulates the HPA axis inside the hippocampus, which provides upstream neuronal feedback on the activity of the HPA axis, and where the expression of the glucocorticoid receptor is altered by 5-HT signaling (26). Serotonergic neurons also innervate the paraventricular nucleus (PVN) of the hypothalamus. Here, the availability of bioactive 5-HT influences the release of the corticotropin-releasing factor [CRF, or corticotropin-releasing hormone (CRH) (27,28)], which in turns stimulates the release of adrenocorticotropic hormone (ACTH) from the pituitary gland. On the side of HPA axis, CRF signaling via CRF type 1 and type 2 receptors as well as the activity of glucocorticoid receptors influence 5-HT synthesis, release and re-uptake (10,29-31). Another way how activity of the HPA axis may alter 5-HT signaling is to impact postsynaptic 5-HT receptors expressed by neurons targeted by serotonergic axons (29,32).
One way to gain insight in how 5-HT neurotransmission works is to eliminate key molecules of serotonergic neurotransmission. Two targets move into the focus of such an experimental approach: the serotonin transporter, which controls the bioactive amount of 5-HT in the brain, and the 5-HT synthesizing enzyme TPH-2. Eliminating TPH-2 results in an experimental model, which deprives an organism of its neuronal 5-HT source, while synthesis of non-neuronal, peripheral 5-HT is sustained by TPH-1. One of the available rodent transgenic TPH-2 knockout mouse lines was generated through homologous recombination of the TPH-2 gene in the 129 R1-ES cell line, establishing a loxP site-flanked exon 5 of the TPH-2 gene. The ES cells were injected in blastocyst of C57BL/6N mice and transplanted into foster mothers. Through breeding the offsprings with a Cre recombinase-expressing mouse, a constitutive TPH-2 knockout (TPH2−/−) was generated (33). In addition to this constitutive knockout model, inducible knockout mouse models that result in a depletion of TPH2 in adult life stages are possible, and multiple constitutive and inducible TPH2−/− mouse lines were established (33-37). With regard to constitutive knockout transgenic animals, TPH2−/− mice seem to share common phenotypes. TPH2−/− males are reduced in growth during development and stay thinner in adulthood, which may be explained by the increased metabolic rate indicated also by increased food and water intake and an increased basal body temperature. Yet experimental data on anxiety-like behaviour seem to differ between laboratories: different transgenic lines display male TPH2−/− mice that are more aggressive than their wildtype littermates. As for the female mice, constitutive TPH2−/− mothers are characterized by low maternal care. Stress-naïve TPH2−/− females displayed low corticosterone metabolites levels in their feces, suggesting hypoactivity of the HPA system under basal conditions due to the lack of 5-HT (38). However, in case of chronic mild stress (CMS), the HPA axis seems to be over activated but adapted to the chronic stress exposure after 3 weeks (38,39). While TPH2−/− females reacted to CMS with an increased corticosterone synthesis, TPH2−/− males remained insensitive to chronic and acute stress. Due to CMS, the expression patterns of the glucocorticoid receptor and mineralocorticoid receptor were differentially, gender-dependently affected in constitutive TPH-2−/− mice in multiple brain regions, including frontal cortex, hippocampus, raphé nuclei and septum [see supplemental data (38)]. Interestingly, serotonin transporter-deficient mice also showed stress-induced alterations in the adrenocortical stress response as measured by corticosterone metabolites excretion levels (40). 5-HT depleted transgenic animals also seem to have disrupted sleep patterns and display altered muscle and bone structure (41,42). While TPH2−/− animals did not show altered pain sensitivity they show an increased fear learning (38). All these phenotypes seen in the constitutive TPH2−/− mice maybe due to putative disturbance of developmental effects caused by neuronal 5-HT depletion. Therefore, the constitutive and the inducible TPH2−/− mice may be applied not only as a rodent model for HPA axis-dependent regulation of HPS cell regulation by 5-HT. They are highly valuable transgenic animal models at hand to investigate to which extend neuronal 5-HT signaling contributes to embryonic development beyond HPS cell generation.
North and colleagues point out that other stress signals engaged during embryonic development, including inflammation or metabolic stress, as well as non-serotonergic neuronal input on the HPA axis may also contribute to or synergistically act on HPS cell generation. In this process, the feedback on the neuronal input given by glucocorticoid signalling may act as a fine-tuning mechanism for homeostatic balance. Based on their findings, they conclude that CNS is at the helm as a master stress response regulator, steering the embryo during detection of and reaction to a number of yet mainly unidentified signals that contribute to HPS cell generation. Finally, the findings of North and colleagues also give an insight into clinical implications as the sustained role of the CNS-mediated HPA axis activity, and thereby regulation of HPS cell generation, may emerge as an important future research field in the context of homeostatic regulation and therapeutic administration. Indeed, and as discussed by North and colleagues, there are findings that show that patients suffering from Cushing’s disease display excessive glucocorticoid levels and major depression, the latter based on dysfunctional 5-HT neurotransmission (43). In addition, the pharmacotherapy of depressed patients with selective serotonin re-uptake inhibitors occasionally caused haematological side effects [for example described in (44)]. As HPA axis-controlled glucocorticoid signalling may not only affect the hematopoietic system, but a broad variety of processes in the human body (Figure 1), this CNS-controlled signalling pathway may contribute to a number of physical disease symptoms observed in stress-related psychiatric diseases. Aside from the implication in psychiatric diseases, the HPA axis also contributes to processing environmental input on developing and established, mature neurons. This epigenetic mechanism has been shown to occur in prenatal stages, to persist throughout adolescence and to be maintained in the adult rodent animal. Since such epigenetic effects are also to occur in human brains, it is of high interest to understand the translational relevance of non-human in vivo and in vitro experimental models, for example as recently discussed for the comparison of adolescent rats and humans in the context of HPA axis and stressors by McCormick et al. (45). Here, human iPS cell-based research models will significantly contribute to translate the findings made in non-human in vivo and in vitro models. The increasing availability and development of differentiation protocols for human iPS cells into genetically and pharmacologically defined cell types of interest will provide a solid starting point for future research. Even more important, as such iPS cell-based models are steadily improved and optimized, they will become of tremendous value to correlate human genetic and physiological characteristics to the observations and discoveries made in animal models. Furthermore, iPS cell-based models may also be established as 3D co-culture or microfluidic cell culture systems (46). The latter provide a microfluidic cell culture model of continuously perfused cell compartments that allow to mimicking physiological processes occurring in the human body and thereby enable to investigate the interaction of human cell types of interest. In combination with findings made in (transgenic) animal models, human iPS cell-based technology provides the potential to provide a significant advance in research areas including the study of human embryonic development, the etiology and pathogenesis of diseases and, finally, the development and improvement of medical treatment.
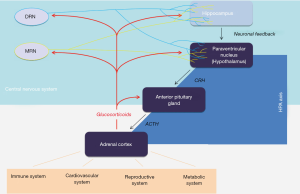
Acknowledgements
Funding: This work was supported by the German Research Foundation (DFG: WA 3446/2-1 to J Waider) and by the H.W. & J. Hector Stiftung (to T Lau).
Footnote
Conflicts of Interest: The authors have no conflicts of interest to declare.
References
- Jacobs BL, Azmitia EC. Structure and function of the brain serotonin system. Physiol Rev 1992;72:165-229. [PubMed]
- Hornung JP. The human raphe nuclei and the serotonergic system. J Chem Neuroanat 2003;26:331-43. [Crossref] [PubMed]
- Dahlström A, Fuxe K. Localization of monoamines in the lower brain stem. Experientia 1964;20:398-9. [Crossref] [PubMed]
- Gaspar P, Lillesaar C. Probing the diversity of serotonin neurons. Philos Trans R Soc Lond B Biol Sci 2012;367:2382-94. [Crossref] [PubMed]
- Lesch KP, Waider J. Serotonin in the modulation of neural plasticity and networks: implications for neurodevelopmental disorders. Neuron 2012;76:175-91. [Crossref] [PubMed]
- Sur C, Betz H, Schloss P. Immunocytochemical detection of the serotonin transporter in rat brain. Neuroscience 1996;73:217-31. [Crossref] [PubMed]
- Buc-Caron MH, Launay JM, Lamblin D, et al. Serotonin uptake, storage, and synthesis in an immortalized committed cell line derived from mouse teratocarcinoma. Proc Natl Acad Sci U S A 1990;87:1922-6. [Crossref] [PubMed]
- Mouillet-Richard S, Mutel V, Loric S, et al. Regulation by neurotransmitter receptors of serotonergic or catecholaminergic neuronal cell differentiation. J Biol Chem 2000;275:9186-92. [Crossref] [PubMed]
- Lau T, Schneidt T, Heimann F, et al. Somatodendritic serotonin release and re-uptake in mouse embryonic stem cell-derived serotonergic neurons. Neurochem Int 2010;57:969-78. [Crossref] [PubMed]
- Lau T, Heimann F, Bartsch D, et al. Nongenomic, glucocorticoid receptor-mediated regulation of serotonin transporter cell surface expression in embryonic stem cell derived serotonergic neurons. Neurosci Lett 2013;554:115-20. [Crossref] [PubMed]
- Matthäus F, Haddjeri N, Sánchez C, et al. The allosteric citalopram binding site differentially interferes with neuronal firing rate and SERT trafficking in serotonergic neurons. Eur Neuropsychopharmacol 2016. [Epub ahead of print]. [Crossref] [PubMed]
- Baudry A, Mouillet-Richard S, Schneider B, et al. miR-16 targets the serotonin transporter: a new facet for adaptive responses to antidepressants. Science 2010;329:1537-41. [Crossref] [PubMed]
- Launay JM, Mouillet-Richard S, Baudry A, et al. Raphe-mediated signals control the hippocampal response to SRI antidepressants via miR-16. Transl Psychiatry 2011;1:e56. [Crossref] [PubMed]
- Mnie-Filali O, Lau T, Matthaeus F, et al. Protein Kinases Alter the Allosteric Modulation of the Serotonin Transporter In Vivo and In Vitro. CNS Neurosci Ther 2016;22:691-9. [Crossref] [PubMed]
- Lau T, Proissl V, Ziegler J, et al. Visualization of neurotransmitter uptake and release in serotonergic neurons. J Neurosci Methods 2015;241:10-7. [Crossref] [PubMed]
- Jacobsen JP, Plenge P, Sachs BD, et al. The interaction of escitalopram and R-citalopram at the human serotonin transporter investigated in the mouse. Psychopharmacology (Berl) 2014;231:4527-40. [Crossref] [PubMed]
- Gaspar P, Nedelec S. Serotonin neurons in a dish. Nat Biotechnol 2016;34:41-2. [Crossref] [PubMed]
- Vadodaria KC, Marchetto MC, Mertens J, et al. Generating human serotonergic neurons in vitro: Methodological advances. Bioessays 2016;38:1123-1129. [Crossref] [PubMed]
- Lu J, Zhong X, Liu H, et al. Generation of serotonin neurons from human pluripotent stem cells. Nat Biotechnol 2016;34:89-94. [Crossref] [PubMed]
- Vadodaria KC, Mertens J, Paquola A, et al. Generation of functional human serotonergic neurons from fibroblasts. Mol Psychiatry 2016;21:49-61. [Crossref] [PubMed]
- Xu Z, Jiang H, Zhong P, et al. Direct conversion of human fibroblasts to induced serotonergic neurons. Mol Psychiatry 2016;21:62-70. [Crossref] [PubMed]
- Kwan W, Cortes M, Frost I, et al. The central nervous system regulates embryonic HPSC production via stress-responsive glucocorticoid receptor signalling. Cell Stem Cell 2016;19:370-82. [Crossref] [PubMed]
- Carroll KJ, North TE. Oceans of opportunity: exploring vertebrate hematopoiesis in zebrafish. Exp Hematol 2014;42:684-96. [Crossref] [PubMed]
- Leonard BE. The HPA and immune axes in stress: the involvement of the serotonergic system. Eur Psychiatry 2005;20 Suppl 3:S302-6. [Crossref] [PubMed]
- Chrousos GP, Kino T. Glucocorticoid signaling in the cell. Expanding clinical implications to complex human behavioral and somatic disorders. Ann N Y Acad Sci 2009;1179:153-66. [Crossref] [PubMed]
- Lai M, McCormick JA, Chapman KE, et al. Differential regulation of corticosteroid receptors by monoamine neurotransmitters and antidepressant drugs in primary hippocampal culture. Neuroscience. 2003;118:975-84. [Crossref] [PubMed]
- Heisler LK, Pronchuk N, Nonogaki K, et al. Serotonin activates the hypothalamic-pituitary-adrenal axis via serotonin 2C receptor stimulation. J Neurosci 2007;27:6956-64. [Crossref] [PubMed]
- Mikkelsen JD, Hay-Schmidt A, Kiss A. Serotonergic stimulation of the rat hypothalamo-pituitary-adrenal axis: interaction between 5-HT1A and 5-HT2A receptors. Ann N Y Acad Sci 2004;1018:65-70. [Crossref] [PubMed]
- Chaouloff F. Serotonin, stress and corticoids. J Psychopharmacol 2000;14:139-51. [Crossref] [PubMed]
- Fairchild G, Leitch MM, Ingram CD. Acute and chronic effects of corticosterone on 5-HT1A receptor-mediated autoinhibition in the rat dorsal raphe nucleus. Neuropharmacology 2003;45:925-34. [Crossref] [PubMed]
- Slotkin TA, Kreider ML, Tate CA, et al. Critical prenatal and postnatal periods for persistent effects of dexamethasone on serotonergic and dopaminergic systems. Neuropsychopharmacology 2006;31:904-11. [Crossref] [PubMed]
- Lanfumey L, Mongeau R, Cohen-Salmon C, et al. Corticosteroid-serotonin interactions in the neurobiological mechanisms of stress-related disorders. Neurosci Biobehav Rev 2008;32:1174-84. [Crossref] [PubMed]
- Gutknecht L, Waider J, Kraft S, et al. Deficiency of brain 5-HT synthesis but serotonergic neuron formation in Tph2 knockout mice. J Neural Transm (Vienna) 2008;115:1127-32. [Crossref] [PubMed]
- Kriegebaum C, Song NN, Gutknecht L, et al. Brain-specific conditional and time-specific inducible Tph2 knockout mice possess normal serotonergic gene expression in the absence of serotonin during adult life. Neurochem Int 2010;57:512-7. [Crossref] [PubMed]
- Kim JY, Kim A, Zhao ZQ, et al. Postnatal maintenance of the 5-Ht1a-Pet1 autoregulatory loop by serotonin in the raphe nuclei of the brainstem. Mol Brain 2014;7:48. [Crossref] [PubMed]
- Alenina N, Kikic D, Todiras M, et al. Growth retardation and altered autonomic control in mice lacking brain serotonin. Proc Natl Acad Sci U S A 2009;106:10332-7. [Crossref] [PubMed]
- Pelosi B, Pratelli M, Migliarini S, et al. Generation of a Tph2 Conditional Knockout Mouse Line for Time- and Tissue-Specific Depletion of Brain Serotonin. PLoS One 2015;10:e0136422. [Crossref] [PubMed]
- Gutknecht L, Popp S, Waider J, et al. Interaction of brain 5-HT synthesis deficiency, chronic stress and sex differentially impact emotional behavior in Tph2 knockout mice. Psychopharmacology (Berl) 2015;232:2429-41. [Crossref] [PubMed]
- Touma C, Palme R, Sachser N. Analyzing corticosterone metabolites in fecal samples of mice: a noninvasive technique to monitor stress hormones. Horm Behav 2004;45:10-22. [Crossref] [PubMed]
- Jansen F, Heiming RS, Lewejohann L, et al. Modulation of behavioural profile and stress response by 5-HTT genotype and social experience in adulthood. Behav Brain Res 2010;207:21-9. [Crossref] [PubMed]
- Mosienko V, Beis D, Pasqualetti M, et al. Life without brain serotonin: reevaluation of serotonin function with mice deficient in brain serotonin synthesis. Behav Brain Res 2015;277:78-88. [Crossref] [PubMed]
- Whitney MS, Shemery AM, Yaw AM, et al. Adult Brain Serotonin Deficiency Causes Hyperactivity, Circadian Disruption, and Elimination of Siestas. J Neurosci 2016;36:9828-42. [Crossref] [PubMed]
- Sonino N, Fava GA. Psychiatric disorders associated with Cushing's syndrome. Epidemiology, pathophysiology and treatment. CNS Drugs 2001;15:361-73. [Crossref] [PubMed]
- Flanagan RJ, Dunk L. Haematological toxicity of drugs used in psychiatry. Hum Psychopharmacol 2008;23 Suppl 1:27-41. [Crossref] [PubMed]
- McCormick MC, Green MR, Simone JJ. Translational relevance of rodent models of hypothalamic-pituitary-adrenal function and stressors in adolescence. Neurobiology of Stress 2016. [Epub ahead of print]. [Crossref]
- Bhatia SN, Ingber DE. Microfluidic organs-on-chips. Nat Biotechnol 2014;32:760-72. [Crossref] [PubMed]
Cite this article as: Waider J, Ziegler J, Lau T. Outside the brain: an inside view on transgenic animal and stem cell-based models to examine neuronal serotonin-dependent regulation of HPA axis-controlled events during development and adult stages. Stem Cell Investig 2016;3:94.