Novel therapeutic approaches: Rett syndrome and human induced pluripotent stem cell technology
Introduction
Human induced pluripotent stem cells (hiPSC)
Induced pluripotent stem cells (iPSC) provide a great platform for therapeutic (regenerative medicine, patient specific personalized medicine, cell replacement therapy) and non-therapeutic applications (disease modeling, drug discovery, pharmaceutical testing and toxicology). The discovery of iPSC from mouse skin fibroblasts using retroviral mediated reactivation of four pluripotent transcription factors (OCT4, SOX2, KLF4, c-MYC) by Takahashi and Yamanaka in 2006. Late in 2007, Thomson and Yu (University of Wisconsin-Madison, USA) and Yamanaka et al. (University of Kyoto, Japan) converted human fibroblasts into hiPSC and revealed the use of two alternative factors (Nanog and Lin 28) to facilitate the programming process using a lentiviral system (1). The unique properties of iPSC, self-renewal and differentiation of three germ layers similar to embryonic stem cells (ESC) are considered as novel cell sources for studying neurodegenerative diseases (2). hiPSCs are promised to develop novel patient-specific cell therapies and research models for inherited and acquired diseases. Neuronal model system differentiation from patient-specific hiPSC to mimic central nervous system (3) is a high-throughput screening platform for novel therapeutic targets and drugs.
An incurable neurological disorder—Rett syndrome (RTT)
RTT (OMIN # 312750), a rare and incurable postnatal neurological disorder predominantly affecting females with an incidence of 1 in 10,000 (4) is characterized by an apparently non- symptomatic phase for the first 6–18 months of age followed by apraxia, deceleration of head growth, gait abnormalities, stereotypic hand movements, and mental retardation. Classical RTT caused by MeCP2 mutations occur predominantly as C > T transitions of CpG dinucleotides mostly on the paternal X chromosome (5). There are more than 300 different mutations found in the MeCP2 gene in which 90% were classical patients. Most of these mutations are found in eight different “hot spots” such as missense or nonsense mutations within the MBD (R106W, R133C, T158M, and R168X) or TRD (R255X, R270X, R294X, and R306C) (6). Small C-terminal deletions, with one or both breakpoints located within the “deleted prone region” (DPR) of exon 4, account for 10% of the cases. Atypical RTT caused by mutations in either cyclin-dependent kinase-like 5 (CDKL5) and netrinG1 (NTNG1) or FOXG1/XBF-1 (7-9). However, at least 5% of typical forms and more other atypical forms are not linked to any of 4 genes known to be involved in the disease. Sudden unexpected death occurs in one-quarter of deaths caused by RTT (10). The course and severity of RTT are determined by the location, type, severity of mutation and X-inactivation. Therefore, two girls of the same age with the same mutation can appear quite different. We already detailed about the basic knowledge on RTT, MeCP2, and hiPSCs in our previous publication (11).
RTT & hiPSC research in India
RTT, the second most common cause of intellectual disability in girls after Down syndrome in girls and women, was first recognized in the 1960s and the first report from India was in 1994 (12). Though RTT has a prevalence of 1 per 10,000 to 22,000 (13) in India, there are very few studies and case reports of RTT in India (14). Some of the studies were detection of two deletions of 44 bp (c.1157_1200del44 or p.L386fs) and 38 bp (c.1151_1188del38 or p.P384fs) in exon 4 or C-terminal segment (CTS) region of MeCP2 in classical RTT patients of Indian origin (15); an unique family carrying non-identical MeCP2 mutations in exon 2 wherein the proband with classical RTT was carrying a de-novo early truncating frameshift mutation while her asymptomatic mother was carrying a missense mutation were both predicted as pathogenic mutations (16); c.1160C > T (P387L) in exon 4 of the MeCP2 gene homozygous mutation in Indian female patient was also reported recently (17). RTT is now recognized as one of the non-curable devastating neurodevelopmental disorders (18) that greatly affect society (19). Approximately, around 50 review articles were published about iPSC in India and a lesser number of research work done in hiPSC especially about characterization, differentiation and reprogramming factors.
There is a holistic therapy for curing the postnatal neurological disorders using the ancient medicinal systems like Siddha and Ayurveda in India. We further referred many recent in-depth reviews on hiPSC and the curative ayurvedic drugs for neurodegenerative disorders mainly for RTT.
Therapeutic target for treating RTT
The MeCP2 gene is important for healthy brain activity. Mutations in MeCP2 gene alter the level of MeCP2 and some downstream factors, which can cause neuronal defects in RTT.
MeCP2-Glutamatergic neurons
There are two types of neurons play a key role in RTT such as inhibitory and excitatory neurons. RTT affects every part of the brain. Generally, excitatory neurons are thought to carry information flow while inhibitory neurons are required for tuning the circuits. Restoring MeCP2 gene expression only in glutamatergic neurons leads to complementary phenotypes of tremor, anxiety, and acoustic startle response (20).
MeCP2-GABAergic neurons
The inhibitory GABAergic neurons results in social problems and repetitive behaviors. Genetically restoring MeCP2 expression only in GABAergic neurons of male MeCP2 null mice enhanced inhibitory signaling, extended lifespan, rescued ataxia, apraxia, and social abnormalities but did not rescue tremor or anxiety. Restoring MeCP2 in neither excitatory nor inhibitory neurons alone is sufficient to fully rescue premature lethality, RTT-like symptoms, and RTT. Hence, modulating the excitatory/inhibitory balance through GABAergic neurons could prove a viable therapeutic option in RTT (21).
MeCP2 in cholinergic neurons
MeCP2 in cholinergic neurons is necessary and sufficient for autonomic cardiac control, thermoregulation, and survival. Targeting the overactive parasympathetic system may be a useful therapeutic strategy to prevent sudden unexpected death in RTT (10).
Downstream gene target
Loss of MeCP2 function in RTT elevates PTP1B levels and thereby impairs insulin signaling and glucose metabolism. PTPN1 encodes PTP1B which is a major metabolic regulator that inhibits insulin signaling by directly dephosphorylating the insulin receptor and IRS1. Therefore, inhibition of PTP1B is a direct target of MeCP2. Animal study by Krishnan et al. 2015 (22) revealed that the small- molecule inhibitors of PTP1B, the difluoromethyl phosphonic acid CPT157633 and the ursolic acid derivative UA0713 act as a potential downstream gene target for RTT (23).
MeCP2 reexpression
RTT shows a significant deficit in neuron-specific K+-Cl- cotransporter 2 (KCC2) expression, resulting in a delayed GABA functional switch from excitation to inhibition. Restoring KCC2 level rescues GABA functional deficits and therefore acts as a downstream gene target to treat RTT (18).
NF-κB pathway
Loss of MeCP2 function leads to upregulation of Irak1 gene, which is downstream of MeCP2 resulting abnormal activation of NF-κB signaling. Macklis et al. in 2016 found the reduction of NF-κB signaling by genes can increase the dendritic complexity of cortical callosal projection neurons (CPN) and extends lifespan in RTT, indicating potential therapeutic strategies of RTT pathogenesis (24).
Clinical trials and drug discovery in RTT
Current therapeutic approaches aim to increase the expression of brain-derived neurotrophic factor (BDNF), a neurotrophin involved in brain development and plasticity to counteract the deficiency in MeCP2. BDNF does not cross the blood brain barrier (BBB), and therefore it has no immediate therapeutic application for RTT patients; however an alternative growth factor, insulin-like growth factor 1 (IGF1), crosses the BBB and shares similar properties and functions with BDNF. Animal research reveals that systemic administration of the active peptide of IGF1 improves physiological behavior and survival in MeCP2 mutant mice suggesting that IGF1 can be a treatment in RTT patients (25). IGF1 can also rescue the impaired KCC2 level as a downstream gene target in RTT. Phase I clinical trials of recombinant IGF-1 treatment in patients with RTT have produced encouraging outcomes leads to ongoing Phase II trials. There are about 40 studies on RTT present in the clinicaltrial.gov, the drug based studies were listed in the below Table 1. There is no drug marketed for treating RTT till now.
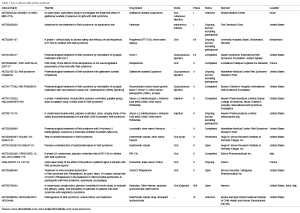
Full table
Current trends in hiPSC technology
Recent advances in stem cell research, especially in the development of iPSC technology, provide a new paradigm for drug screening by permitting the use of human cells with the same genetic makeup as the patients without the typical quantity constraints associated with patient primary cells (26). The delivery of iPS cells treated with mitomycin C (MMC) loading with gold nanorods (AuNRs) for the targeted photothermal treatment of gastric cancer resulted in killing the tumor cells by the heat generated from the gold nanorods. This suggested that pre-treated iPS cells with MMC can be used as a novel and safe approach for targeted tumor therapy (27). Nanoplatform by the combination of Au nanorods, SiO2, CXCR4 nanoparticles with hiPSC for the photothermal treatment paves a great promise for clinical translation in the near future (28). hiPSCs can be used as an autologous source for cell therapy. Transplantation of human iPSC-derived cells is a safe and efficient approach to promoting recovery after stroke and can be used to supply the injured brain with new neurons for replacement (29). Furthermore, the ability to simulate organ systems with hiPSCs may allow for studying the effects of a drug’s metabolites on target and non-target cell types. This is an important part of a drug’s side effect profile that can currently be observed only in animal models or clinical trials. hiPSCs plays a larger role in studying the effects of polypharmacy, both in the general population and specific patient. Recently, “tissue chip” devices have become prominent because these cellular models are designed to recapitulate the structure and function of human organs, such as the lung, liver and heart; therefore, they are often called “organs-on-a-chip” or “human-on-a-chip” (30). Three-dimensional model systems are expected to be able to mimic human physiology more accurately than traditional two-dimensional cultures, among which “organ-on-a-chip” and “body-on-a-chip” are advanced formats of “tissue-on-a-chip” devices (31). Once “tissue-on-a-chip” devices are developed and accepted as a standard part of the preclinical drug development process, they can be used to predict whether a drug candidate is safe or toxic to humans in the safety of the laboratory setting (32). This is an increasingly important aspect of drug development, taking into account the commonality of multiple medication regimens and a patient population that will likely increase the number of medications as they age (33). A total of 1,500 compounds were tested on wild-type hiPSC-derived neural progenitor cells for their effect on the Wnt/β-catenin signaling pathway for identifying a potential novel neuropsychiatric drug (34).
Limitations associated with drug screening for RTT
Drug screening for neurological disorders is a tedious process and it is insignificant to confirm the efficacy of the respective drug dosage. The main issue is the difference between the mice and human neurons activity against the drug. Drug treatment using hiPSC derived neuron model system is more successful for neurodevelopmental disorders than late-onset neurodegenerative disorders, likely because of the foetal-like properties of the cells (35). It is difficult to correlate the drug pharmacodynamics in the neurodegenerative disorder animal models. There are also some limitations associated with drug screening even in hiPSC such as genetic aberration and teratoma formation by retroviral or lentiviral systems of gene incorporation in the generation of iPSCs. Five oncogenes were found to overexpress in iPSCs whereas the oncogene RAB25 was found to express in cells derived from iPSCs (36). Apart from these limitations, generation of patient specific or mutation specific hiPSC model system is a great challenge and cost effective but ultimately it can be the immortal modal system as compared with animal models. It is unlikely that hiPSC technology will successfully model for all disorders (37).
Indian traditional ayurvedic system: curative drugs for neurological disorders
Ayurveda is a science of life and science of longevity to health and personalized medicine. India is known for its traditional medicinal systems—Ayurveda, Siddha, and Unani. Ayurveda has an extensive pharmacopeia, predominantly herbs and minerals. The Ayurvedic concept appeared and developed between 2,500 and 500 BC in India, which is the largest producer of medicinal plants. There are currently about 20,000 medicinal plants have been recorded. Clinical trials are ongoing in more than 100 natural product derived drugs and at least 100 molecules/compounds are in the preclinical development stage (38). In the Indian system of medicine, the following medicinal plants have shown promising activity in neuropsychopharmacology: Allium sativum, Bacopamonnierae, Centellaasiatica, Celastruspaniculatus, Nicotianatabaccum, Withaniasomnifera, Ricinuscommunis, Salvia officinalis, Ginkgo biloba, Huperizaserrata, Angelica sinensis, Uncariatomentosa, Hypericumperforatum, Physostigmavenosum, Acorus Calamus, Curcuma longa, Terminaliachebula, Crocus sativus, Enhydrafluctuans, Valerianawallichii, Glycyrrhizaglabra etc. (39). The Medhya Rasayanas are group of medicinal plants described in Ayurveda, known to improve mental health, intellect ability, immunity and hence longevity. Medha means intellect/retention and Rasayana means therapeutic procedure. Medhya Rasayana is a group of 4 medicinal plants that can be used singly or in combinations.
- Mandukaparni (Centella asiatica). Useful in treating mental retardation (improvement in performance IQ), Social Quotient, immediate memory span.
- Yashtimadhu (Glycyrrhiza glabra). Spatial learning, preliminary free radical scavenging, cerebral ischemia and antioxidant capacity towards LDL oxidation. It increases the circulation into the CNS system, improves learning and memory on scopolamine-induced dementia.
- Guduchi (Tinospora cordifolia). Strong free radical scavenging properties against reactive oxygen and nitrogen species. It is useful for treatment of improving behavior disorders, mental deficit and IQ levels.
- Shankhpushpi (Convolvulus pluricaulis). Anxiolytic and memory enhancing, mood elevating, retard brain aging (40).
Phytochemical based antioxidants may have neuroprotective (preventing apoptosis) and neuroregenerative roles, by reducing or reversing cellular damage and by slowing progression of neuronal cell loss. There is ample scientific and empirical evidence supporting the use of antioxidants for the control of neurological disorders. As the focus of medicine shifts from the treatment of manifest disease to prevention, herbal medicine is coming into consideration, being a renaissance of age-old human tradition (41).
Projected curative drugs for RTT: Ayurveda with hiPSC technology
Medhya Rasayana will cure the symptoms of RTT. The treatment is cost effective and devoid of side effects. As so far the drugs tested for RTT using hiPSC neuronal modal system were having action on synapse number, spine number and soma size. The role of ‘Medhya drugs’ in neuronal stem cells differentiation is also described earlier (42). Earlier reports indicate that ‘Rasayan drugs’ could be used in stem cell therapy based on the regeneration and cell renewal properties. A tablet prepared from four Medhya Rasayana herbs aid in yielding concentrated medicament with the same efficacy as per the classically proposed drug dosage at lower dose (43). The active compounds in Medhya drugs will reverse neurological disorders and psychomotor activities (40). Hence, these active compounds pave a way to cure the RTT symptoms like repetitive hand movements and mental impairment. Figure 1 depicts the drugs tested and projected Madhya drugs need to test in the hiPSC-RTT neuronal model system.
Way forward and conclusions
Comprehensive research on the discovery of novel neuroprotective drug candidates has proven that natural products, such as plant extracts and their bioactive compounds, can have tremendous potential as lead neuroprotective candidates. A source of herb-based drugs severely compromises proper understanding of Ayurveda, which insists on restoring balance to doshas and dhatus, and preventing a repetition of their vitiation, as the first priority. Identification and characterization of new medicinal plants to cure neurological diseases and brain injuries are the major and increasing scientific interest in recent years. There are more than 120 traditional medicines that are being used for the therapy of central nervous system (CNS) disorders in Asian countries (49). Solely using herbal drugs is against the ethos of Ayurveda. Sadly, even in India, current research and practice of Ayurveda are moving on the lines of allopathy where drugs take center stage. Unfortunately, modern medicine based psychoactive drugs have met with limited success in the treatment of various neurological and psychiatric disorders due to multi-factorial nature of these diseases. The world is trying to move towards holistic and integrative approaches, which represent the core of Ayurveda. Using hiPSCs in phase I clinical trials may provide a more sensitive assay for a candidate drug’s toxicity and safety compared with conventional clinical trial phases. Hence, in future the integrative approach of novel drug discovery from Ayurveda using hiPSC will pave the wave for drugging the undruggable diseases.
Acknowledgements
The author would like to thank the Human Genetics Laboratory, Bharathiar University, India for providing necessary infrastructure facilities to conduct this article.
Footnote
Conflicts of Interest: The authors have no conflicts of interest to declare.
References
- Chou BK, Gu H, Gao Y, et al. A facile method to establish human induced pluripotent stem cells from adult blood cells under feeder-free and xeno-free culture conditions: a clinically compliant approach. Stem Cells Transl Med 2015;4:320-32. [Crossref] [PubMed]
- Li W, Chen S, Li JY. Human induced pluripotent stem cells in Parkinson's disease: A novel cell source of cell therapy and disease modeling. Prog Neurobiol 2015;134:161-77. [Crossref] [PubMed]
- Marchetto MC, Carromeu C, Acab A, et al. A model for neural development and treatment of Rett syndrome using human induced pluripotent stem cells. Cell 2010;143:527-39. [Crossref] [PubMed]
- Dunn HG, MacLeod PM. Rett syndrome: review of biological abnormalities. Can J Neurol Sci 2001;28:16-29. [PubMed]
- Trappe R, Laccone F, Cobilanschi J, et al. MECP2 mutations in sporadic cases of Rett syndrome are almost exclusively of paternal origin. Am J Hum Genet 2001;68:1093-101. [Crossref] [PubMed]
- Schanen C, Houwink EJ, Dorrani N, et al. Phenotypic manifestations of MECP2 mutations in classical and atypical Rett syndrome. Am J Med Genet A 2004;126A:129-40. [Crossref] [PubMed]
- Patriarchi T, Amabile S, Frullanti E, et al. Imbalance of excitatory/inhibitory synaptic protein expression in iPSC-derived neurons from FOXG1(+/-) patients and in foxg1(+/-) mice. Eur J Hum Genet 2016;24:871-80. [Crossref] [PubMed]
- Das DK, Jadhav V, Ghattargi VC, et al. Novel mutation in forkhead box G1 (FOXG1) gene in an Indian patient with Rett syndrome. Gene 2014;538:109-12. [Crossref] [PubMed]
- Ricciardi S, Ungaro F, Hambrock M, et al. CDKL5 ensures excitatory synapse stability by reinforcing NGL-1-PSD95 interaction in the postsynaptic compartment and is impaired in patient iPSC-derived neurons. Nat Cell Biol 2012;14:911-23. [Crossref] [PubMed]
- Herrera JA, Ward CS, Wehrens XH, et al. Methyl-CpG binding-protein 2 function in cholinergic neurons mediates cardiac arrhythmogenesis. Hum Mol Genet 2016;25:4983-95. [PubMed]
- Balachandar V, Dhivya V, Gomathi M, et al. A review of Rett syndrome (RTT) with induced pluripotent stem cells. Stem Cell Investig 2016;3:52. [Crossref] [PubMed]
- Kalra V, Sud DT. Rett syndrome. Indian Pediatr 1994;31:711-5. [PubMed]
- Jellinger KA. Rett Syndrome -- an update. J Neural Transm (Vienna) 2003;110:681-701. [Crossref] [PubMed]
- Kumar S, Alexander M, Gnanamuthu C. Recent experience with Rett syndrome at a tertiary care center. Neurol India 2004;52:494-5. [PubMed]
- Khajuria R, Sapra S, Ghosh M, et al. Novel human pathological mutations. Gene symbol: MECP2. Disease: Rett Syndrome. Hum Genet 2010;127:117-8.
- Khajuria R, Gupta N, Sapra S, et al. A novel MECP2 change in an indian boy with variant rett phenotype and congenital blindness: implications for genetic counseling and prenatal diagnosis. J Child Neurol 2011;26:209-13. [Crossref] [PubMed]
- Bhanushali AA, Mandsaurwala A, Das BR. Homozygous c.1160C>T (P38L) in the MECP2 gene in a female Rett syndrome patient. J Clin Neurosci 2016;25:127-9. [Crossref] [PubMed]
- Tang X, Kim J, Zhou L, et al. KCC2 rescues functional deficits in human neurons derived from patients with Rett syndrome. Proc Natl Acad Sci U S A 2016;113:751-6. [Crossref] [PubMed]
- Jung YW, Hysolli E, Kim KY, et al. Human induced pluripotent stem cells and neurodegenerative disease: prospects for novel therapies. Curr Opin Neurol 2012;25:125-30. [Crossref] [PubMed]
- Meng X, Wang W, Lu H, et al. Manipulations of MeCP2 in glutamatergic neurons highlight their contributions to Rett and other neurological disorders. Elife 2016;5:e14199. [Crossref] [PubMed]
- Ure K, Lu H, Wang W, et al. Restoration of Mecp2 expression in GABAergic neurons is sufficient to rescue multiple disease features in a mouse model of Rett syndrome. Elife 2016;5:e14198. [Crossref] [PubMed]
- Krishnan N, Krishnan K, Connors CR, et al. PTP1B inhibition suggests a therapeutic strategy for Rett syndrome. J Clin Invest 2015;125:3163-77. [Crossref] [PubMed]
- Tautz L. PTP1B: a new therapeutic target for Rett syndrome. J Clin Invest 2015;125:2931-4. [Crossref] [PubMed]
- Kishi N, MacDonald JL, Ye J, et al. Reduction of aberrant NF-κB signalling ameliorates Rett syndrome phenotypes in Mecp2-null mice. Nat Commun 2016;7:10520. [Crossref] [PubMed]
- Pini G, Scusa MF, Congiu L, et al. IGF1 as a Potential Treatment for Rett Syndrome: Safety Assessment in Six Rett Patients. Autism Res Treat 2012;2012:679801. [Crossref] [PubMed]
- Xu XH, Zhong Z. Disease modeling and drug screening for neurological diseases using human induced pluripotent stem cells. Acta Pharmacol Sin 2013;34:755-64. [Crossref] [PubMed]
- Yang M, Liu Y, Hou W, et al. Mitomycin C-treated human-induced pluripotent stem cells as a safe delivery system of gold nanorods for targeted photothermal therapy of gastric cancer. Nanoscale 2017;9:334-340. [Crossref] [PubMed]
- Liu Y, Yang M, Zhang J, et al. Human Induced Pluripotent Stem Cells for Tumor Targeted Delivery of Gold Nanorods and Enhanced Photothermal Therapy. ACS Nano 2016;10:2375-85. [Crossref] [PubMed]
- Oki K, Tatarishvili J, Wood J, et al. Human-induced pluripotent stem cells form functional neurons and improve recovery after grafting in stroke-damaged brain. Stem Cells 2012;30:1120-33. [Crossref] [PubMed]
- Luni C, Serena E, Elvassore N. Human-on-chip for therapy development and fundamental science. Curr Opin Biotechnol 2014;25:45-50. [Crossref] [PubMed]
- Skardal A, Shupe T, Atala A. Organoid-on-a-chip and body-on-a-chip systems for drug screening and disease modeling. Drug Discov Today 2016;21:1399-411. [Crossref] [PubMed]
- Hosoya M, Czysz K. Translational Prospects and Challenges in Human Induced Pluripotent Stem Cell Research in Drug Discovery. Cells 2016;5:E46. [Crossref] [PubMed]
- Ko HC, Gelb BD. Concise review: drug discovery in the age of the induced pluripotent stem cell. Stem Cells Transl Med 2014;3:500-9. [Crossref] [PubMed]
- Zhao WN, Cheng C, Theriault KM, et al. A high-throughput screen for Wnt/β-catenin signaling pathway modulators in human iPSC-derived neural progenitors. J Biomol Screen 2012;17:1252-63. [Crossref] [PubMed]
- Rajamohan D, Matsa E, Kalra S, et al. Current status of drug screening and disease modelling in human pluripotent stem cells. Bioessays 2013;35:281-98. [Crossref] [PubMed]
- Singh VK, Kalsan M, Kumar N, et al. Induced pluripotent stem cells: applications in regenerative medicine, disease modeling, and drug discovery. Front Cell Dev Biol 2015;3:2. [Crossref] [PubMed]
- Maury Y, Gauthier M, Peschanski M, et al. Human pluripotent stem cells for disease modelling and drug screening. Bioessays 2012;34:61-71. [Crossref] [PubMed]
- Chopra A, Saluja M, Tillu G. Ayurveda-modern medicine interface: A critical appraisal of studies of Ayurvedic medicines to treat osteoarthritis and rheumatoid arthritis. J Ayurveda Integr Med 2010;1:190-8. [Crossref] [PubMed]
- Kumar GP, Khanum F. Neuroprotective potential of phytochemicals. Pharmacogn Rev 2012;6:81-90. [Crossref] [PubMed]
- Ray S, Ray A. Medhya Rasayanas in Brain Function and Disease. Med chem 5:505-511.
- Bizimenyera ES, Aderogba MA, Eloff JN, et al. Potential of neuroprotective antioxidant-based therapeutics from Peltophorum africanum Sond. (Fabaceae). Afr J Tradit Complement Altern Med 2006;4:99-106. [PubMed]
- Joshi KS, Bhonde R. Insights from Ayurveda for translational stem cell research. J Ayurveda Integr Med 2014;5:4-10. [Crossref] [PubMed]
- Reena K, Abhimanyu K, Kumar KN. Formulation and standardization of Medhya Rasayana–A novel Ayurvedic compound nootropic drug. Pharmacognosy Journal 2013;5:72-6. [Crossref]
- Dhingra D, Valecha R. Evaluation of the antidepressant-like activity of Convolvulus pluricaulis choisy in the mouse forced swim and tail suspension tests. Med Sci Monit 2007;13:BR155-61. [PubMed]
- Stough C, Lloyd J, Clarke J, et al. The chronic effects of an extract of Bacopa monniera (Brahmi) on cognitive function in healthy human subjects. Psychopharmacology (Berl) 2001;156:481-4. [Crossref] [PubMed]
- Holcomb LA, Dhanasekaran M, Hitt AR, et al. Bacopa monniera extract reduces amyloid levels in PSAPP mice. J Alzheimers Dis 2006;9:243-51. [PubMed]
- Saraf MK, Prabhakar S, Khanduja KL, et al. Bacopa monniera Attenuates Scopolamine-Induced Impairment of Spatial Memory in Mice. Evid Based Complement Alternat Med 2011;2011:236186. [Crossref] [PubMed]
- Kuboyama T, Tohda C, Zhao J, et al. Axon- or dendrite-predominant outgrowth induced by constituents from Ashwagandha. Neuroreport 2002;13:1715-20. [Crossref] [PubMed]
- Kumar V. Potential medicinal plants for CNS disorders: an overview. Phytother Res 2006;20:1023-35. [Crossref] [PubMed]
Cite this article as: Gomathi M, Balachandar V. Novel therapeutic approaches: Rett syndrome and human induced pluripotent stem cell technology. Stem Cell Investig 2017;4:20.