Role of “osteogenic” cardiac fibroblasts in pathological heart calcification
Calcification of mammalian soft tissues as induced by age and injury is a cell mediated process that resembles bone formation in the skeletal system with calcification of the extracellular matrix by cells capable of mineralization (1). The abnormal accumulation of calcium salts occurs in the pathologic calcification of any tissue (2). Two basic forms of calcification are recognized; dystrophic and metastatic (3). Ischemic, traumatic, infectious and/or inflammatory, and neoplastic processes among others are significant causes of dystrophic myocardial calcification. Any abnormality of calcium metabolism can lead to metastatic myocardial calcification, including chronic renal failure, bone destruction or increased bone turnover, hyperparathyroidism, and vitamin D-related disorders. Calcification of the cardiovascular system, such as aortic valve and vascular calcification, has important clinical significance (4). Myocardial calcification is an unusual form of soft tissue calcification characterized by abnormal calcific accumulation within the heart muscle which can occur in the absence of calcification of vessels, valves, or other organs (5). Calcification within the myocardium is one of the most common underlying causes of heart block where calcification and fibrosis of the conduction system interrupts smooth propagation of electrical impulses (6). The occurrence of heart muscle calcification is observed in senile heart diseases and in varieties of disease conditions, such as chronic renal disease, diabetes, and myocardial injury secondary to inflammation or ischemia (7). However, the identity of cells and the mechanism contributing to pathological myocardial calcification has remained elusive.
The heart is composed of cardiomyocytes (CMs) as well as interstitial fibroblasts in addition to other cells. The uninjured adult murine myocardium is comprised of roughly 56% myocytes, 27% fibroblasts, 7% endothelial cells and 10% vascular smooth muscle cells as well as other immune cells (8). Studies on the heart have historically focused in main on CMs, but the role of cardiac fibroblasts (CFs) has received increasing recent attention as pivotal functions in the maintenance of cardiac function, physiological cardiac remodeling after heart stress and pathological remodeling (9). CFs can exhibit plasticity after heart injury and adopt alternate cell fates (10). We have recently demonstrated that ataxia telangiectasia mutated (ATM)-regulated effects within CFs are pivotal in doxorubicin-induced cardiotoxicity, and antagonism of ATM and its functions may have potential therapeutic implications (11). Ectopic calcification of soft tissues usually occurs in areas of injury associated with fibrosis (12). CFs adopt osteogenic cell fate which are thought to contribute to pathological cardiac calcification, as illustrated in the paper by Pallai et al. in the recent issue of Cell Stem Cell (Figure 1) (13).
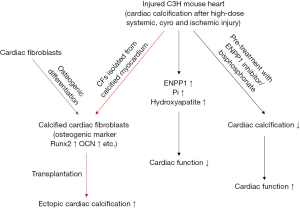
These authors started from the observation that deposition of calcium hydroxyapatite (visualized with Alizarin Red staining) and induction of expression of stable canonical osteoblast markers (i.e., Runx2, osteocalcin quantified by qPCR) were confirmed in CFs following osteogenic differentiation or CFs isolated from multiple models of myocardial calcification induced after a variety of injuries (i.e., high-dose systemic steroids, cyro and ischemic injury), suggesting that CFs can adopt an osteoblast cell-like fate in vitro and in vivo. Next, they observed that CFs adjacent to calcified myocardium induced by injury not only expressed abundant osteogenic markers (i.e., Runx2, osteocalcin) and extracellular matrix protein (i.e., osteopontin), but also CFs could directly influence mineralization of extracellular matrix. Moreover, cell transplantation experiments showed that CFs harvested following explant culture of calcified or uninjured myocardium of C3H mice when injected into subcutaneous pockets of another host showed a direct role of CFs from calcific heart lesions in mediating ectopic soft tissue calcification.
They then addressed mechanisms of osteogenesis or mineralization with RNA-seq on uninjured and injured cardiac regions of C3H strains (calcification after injury) and B6 strains (no calcification after injury) and identified that 11 osteogenic genes (ENPP1, Runx2, Col1a1, and Fibronectin etc.) were markedly higher in injured C3H hearts compared to control uninjured C3H hearts or injured B6 hearts. None of the B6 mice after injury developed cardiac calcification, therefore the disparate injury response in C3H and B6 mouse strains demonstrated a mechanism that is differentially regulated in calcific versus non-calcific hearts.
ENPP1 is expressed in differentiated osteoblasts, is thought to be an established mediator of osteoblast maturation and tissue mineralization by increasing extracellular pyrophosphate, and animals deficient in ENPP1 have decreased mineralization of long bones (14). What is the role of ENPP1 in heart calcification? To demonstrate its causal involvement, Pallai et al. first showed abundant expression of ENPP1 in CFs and significantly higher phosphate (Pi) levels in calcified regions of the heart after injury. They also used Raman spectroscopy (15) to show that extracellular pyrophosphate (PPi) generated by ENPP1 can induce myocardial calcific deposits by serving as a substrate for Pi generation and formation of calcium hydroxyapatite. Mechanistically, they provide evidence that CFs harvested from calcific myocardium are sufficient to induce mineralization of the matrix via upregulation of ENPP1.
They next investigated involvement of the ENPP1-PPi-Pi axis as a target to decrease ectopic cardiac calcification. They provide evidence that post-injury cardiac calcification was decreased by pre-treatment with the ENPP1 inhibitors SYL-001 or ARL67156, respectively. Moreover, pre-treatment with inhibitors of bone mineralization, bisphosphonates (e.g., etidronate) which serve as functional antagonists of the ENPP1-PPi-Pi axis, followed by injury could significantly attenuate calcification and improve cardiac function. However, etidronate administered after the development of calcification did not reverse or decrease the amount of deposited calcium. These findings highlight the aberrant plasticity of CFs in contributing to ectopic calcification and identify novel pharmacological targets (CF and the ENPP1-PPi-Pi axis) for therapeutic implications.
The cell-matrix mechanics described in this study are similar to that of underlying vascular and valvular calcification. Vascular cells, like bone cells, interact with their matrix substrate through molecular signals and biomechanical signals to control pathological calcification of the artery wall and cardiac valves (4). The present study by Pallai et al. also showed involvement of cell-matrix mechanics in pathological heart calcification—that CFs express ENPP1, and ENPP1-generated PPi in heart increases mineralization via hydrolysis of PPi to generate Pi by tissue non-specific alkaline phosphatase (TANP) and subsequent calcium hydroxyapatite formation in extracellular matrix to develop post-injury cardiac calcification. Calcification of the extracellular matrix is critically regulated by the balance of extracellular Pi and PPi via regulation of ENPP1. The interaction of CFs with matrix may contribute to control of pathological cardiac calcification. The authors add to our understanding of CF-matrix mechanics in pathological heart calcification, but it is necessary to further investigate the interaction of CM-matrix or CF-CM.
The study by Pallai et al. is the first to focus on the identity of cells contributing to pathological heart calcification. Their results showed that, surprisingly, CFs and not CMs, as would be expected a priori, adopt osteogenic fates and that the ENPP1-PPi-Pi axis is a potential cellular and pharmacological target for developing therapies for pathological heart calcification. Per-administration of small molecules (SYL-001 or ARL67156) that inhibit ENPP1, or inhibitors of bone mineralization, bisphosphonates (e.g., etidronate), that inhibit the function of the ENPP1-PPi-Pi axis followed by injury, led to significantly decreased cardiac calcification and preservation of post-injury cardiac function in the present study, and might serve as a potential anti-calcification drug. Cell-type specific targeted therapy (e.g., fibroblast-specific ENPP1 antagonism), possibly in combination with those targeting other pathways involved in injury-induced cardiac calcification might pose further therapeutic possibilities which need to be addressed in translational studies targeting lineage-restricted modulation of cell functions.
Acknowledgements
This work is supported in part by the Japan Agency for Medical Research and Development (AMED) and Grants-in-Aid for Scientific Research from the Ministry of Education, Culture, Sports, Science and Technology of Japan.
Footnote
Conflicts of Interest: The authors have no conflicts of interest to declare.
References
- Demer LL, Tintut Y. Inflammatory, metabolic, and genetic mechanisms of vascular calcification. Arterioscler Thromb Vasc Biol 2014;34:715-23. [Crossref] [PubMed]
- Nance JW Jr, Crane GM, Halushka MK, et al. Myocardial calcifications: pathophysiology, etiologies, differential diagnoses, and imaging findings. J Cardiovasc Comput Tomogr 2015;9:58-67. [Crossref] [PubMed]
- Perkins JA. Tissue Renewal, Regeneration, and Repair. In: Perkins JA, editor. Robbins and Cotran Pathologic Basis of Disease. Eighth Edition. Philadelphia: WB Saunders; 2010;79e110.
- Hsu JJ, Lim J, Tintut Y, et al. Cell-matrix mechanics and pattern formation in inflammatory cardiovascular calcification. Heart 2016;102:1710-5. [Crossref] [PubMed]
- Shackley BS, Nguyen TP, Shivkumar K, et al. Idiopathic massive myocardial calcification: a case report and review of the literature. Cardiovasc Pathol 2011;20:e79-83. [Crossref] [PubMed]
- Lev M. Anatomic Basis for Atrioventricular Block. Am J Med 1964;37:742-8. [Crossref] [PubMed]
- Rostand SG, Sanders C, Kirk KA, et al. Myocardial calcification and cardiac dysfunction in chronic renal failure. Am J Med 1988;85:651-7. [Crossref] [PubMed]
- Banerjee I, Fuseler JW, Price RL, et al. Determination of cell types and numbers during cardiac development in the neonatal and adult rat and mouse. Am J Physiol Heart Circ Physiol 2007;293:H1883-91. [Crossref] [PubMed]
- Fujiu K, Nagai R. Contributions of cardiomyocyte-cardiac fibroblast-immune cell interactions in heart failure development. Basic Res Cardiol 2013;108:357. [Crossref] [PubMed]
- Ubil E, Duan J, Pillai IC, et al. Mesenchymal-endothelial transition contributes to cardiac neovascularization. Nature 2014;514:585-90. [Crossref] [PubMed]
- Zhan H, Aizawa K, Sun J, et al. Ataxia telangiectasia mutated in cardiac fibroblasts regulates doxorubicin-induced cardiotoxicity. Cardiovasc Res 2016;110:85-95. [Crossref] [PubMed]
- Pugashetti R, Shinkai K, Ruben BS, et al. Calcium may preferentially deposit in areas of elastic tissue damage. J Am Acad Dermatol 2011;64:296-301. [Crossref] [PubMed]
- Pillai IC, Li S, Romay M, et al. Cardiac Fibroblasts Adopt Osteogenic Fates and Can Be Targeted to Attenuate Pathological Heart Calcification. Cell Stem Cell 2017;20:218-232.e5. [Crossref] [PubMed]
- Johnson K, Goding J, Van Etten D, et al. Linked deficiencies in extracellular PP(i) and osteopontin mediate pathologic calcification associated with defective PC-1 and ANK expression. J Bone Miner Res 2003;18:994-1004. [Crossref] [PubMed]
- Chen KH, Li MJ, Cheng WT, et al. Identification of monoclinic calcium pyrophosphate dihydrate and hydroxyapatite in human sclera using Raman microspectroscopy. Int J Exp Pathol 2009;90:74-8. [Crossref] [PubMed]
Cite this article as: Zhan H, Suzuki T. Role of “osteogenic” cardiac fibroblasts in pathological heart calcification. Stem Cell Investig 2017;4:26.