Indoleamine 2,3-dioxygenase and survivin peptide vaccine combined with temozolomide in metastatic melanoma
Introduction
Peptide vaccination as therapy of cancer is able to induce robust CD8+ T-cell responses towards a multitude of antigens. However, despite multiple clinical trials, no peptide vaccines have yet reached approval for the treatment of human cancers. As reviewed by Aranda et al. (1), this might be due to low antigenicity of cancer cells, insufficient immunogenicity of the peptide vaccines, and the immunosuppressive mechanism of cancer. To overcome the challenges associated with inducing clinical responses by peptide vaccination, one approach is to combine vaccination with other immune stimulatory treatments for augmented effect. Combining immunotherapy with chemotherapy may seem counterintuitive as cells of the immune system are sensible to chemotherapy, but recent studies indicate an immune component of chemotherapy’s anti-tumoral effect (2). In Denmark, temozolomide (TMZ) is used as a standard chemotherapy in treatment of malignant melanoma (MM) and the lymphodepleting effect of TMZ has been correlated with clinical response in MM. Furthermore, we have previously shown that TMZ induces a shift from naïve to differentiated CD8+ T-cells and induces expansion of tumor associated antigen (TAA) specific CD8+ T-cells (3). TMZ has been tested in combination with other immunotherapies including interferon-α and IL-2 without increased clinical benefit (4,5).
In this study, we combined a human leukocyte antigen (HLA)-A2 specific peptide vaccine with epitopes from indoleamine 2,3-dioxygenase (IDO) and from survivin with TMZ. HLA-A2 was chosen as it is the most frequent tissue types expressed in melanoma patients (6). IDO is an enzyme that facilitates the degradation of tryptophan to kynurenine. In 2003, Uyttenhove et al. (7) linked expression of IDO to tumoral immune resistance, and later on IDO’s immune inhibitory ability was coupled to several mechanisms: depriving T-cells of tryptophan initiates a GCN2 kinase mediated stress response resulting in proliferative arrest and anergy (8); inducing apoptosis by increasing the concentrations of kynurenine and inducing conversion of T helper cells (Th) 17 into T-regulatory T-cells (Treg) (9). In cancer patients, clusters of CD8+ cytotoxic T-lymphocyte (CTL) and CD4+ Th cells spontaneously recognizing peptides derived from IDO have been observed (10,11). These findings led us to initiate a vaccination trial for lung cancer patients using an IDO-derived peptide which resulted in two ongoing clinical responders and IDO specific CD8+ T-cell immunity in all patients (12).
Survivin is an anti-apoptotic protein first discovered in 1997 (13). It is overexpressed in a variety of cancers including MM (13,14) and its expression has been correlated to a poor prognosis and disease progression in, e.g., colon cancer and neuroblastoma (15,16). Survivin is expressed in a subpopulation of cancer cells with a high proliferative potential and as a consequence, targeting survivin means targeting tumor cells with the highest proliferative rate (17). Specific T-cells able to recognize a survivin-derived peptide have been observed both in in vitro and in clinical studies (18,19).
When treating MM patients with a peptide vaccine with survivin and IDO in combination with TMZ, we aimed for an increased clinical benefit by targeting several immune inhibiting mechanisms in the tumor microenvironment as well as directly targeting the malignant cells.
Methods
Study design
Patients were enrolled in this phase II study at Herlev Hospital, Capital Region, Denmark. Major inclusion criteria were histological verified MM, metastatic disease, evaluable disease according to Response Evaluation Criteria In Solid Tumors (RECIST 1.1), a performance status equal to or lower than 2 according to Eastern Cooperative Oncology Group (ECOG) (20) and adequate hematological function. Major exclusion criteria were other malignant diseases, use of immune suppressive medication, severe medical disorders, infections, severe allergy and active autoimmune diseases. For a complete description of inclusion and exclusion criteria, we refer to www.clinicaltrials.gov (NCT01543464). If the patients met all criteria, they were tested for HLA-A2 tissue type. Only HLA-A2 positive patients received experimental treatment with the vaccine in combination with oral TMZ. HLA-A2 negative patients would receive standard therapy with oral TMZ. The study was approved by Danish Health and Medicines Agency and the local Ethics Committee, Capital Region of Denmark and conducted in accordance with the Helsinki II declaration and good clinical practice (GCP). The study is registered at www.clinicaltrials.gov (NCT01543464) and https://eudract.ema.europa.eu/ (EudraCT #2011-004577-91). Primary endpoint was clinical benefit rate (CBR) defined as complete responses (CR) plus partial responses (PR) and stable disease (SD) for at least 6 months. Secondary endpoint was immunological response and tertiary endpoint was toxicity. The planned number of patients to be treated was 30. However, due to changes in standard treatment, algorithm for metastatic melanoma accrual to the trial became increasingly difficult and it was therefore decided to close the trial before full enrollment.
Treatment
HLA-A2 patients were treated with 150 mg/m2 TMZ daily for 7 days, biweekly, followed by subcutaneous (sc) vaccination with IDO5 and Sur1M2 peptide in Montanide solution at day 8. Topical 5% imiquimod cream (Medea, Alleröd, Denmark) was applied to the vaccine-site and kept under an occlusive bandage 6–12 hours before injection. Ten minutes prior to vaccination, patients received an injection of 75 µg granulocyte-macrophage colony-stimulating factor (GM-CSF) at the intended site of vaccine administration (Berlex Laboratories, Inc., USA).
Treatment was repeated biweekly for a period of up to 6 months. Patients still benefiting from treatment would continue TMZ biweekly and vaccination injections every 4 weeks.
Peptide vaccine
The vaccine consists of a 9-amino acid long peptide from survivin denoted Sur1M2, one-letter amino acid code LMLGEFLKL, and a 9-amino acid long peptide from IDO denoted IDO5, one letter amino acid code ALLEIASCL (Polypeptide Laboratories, France SAS). The 250 µg of each peptide were dissolved in 2% dimethyl sulfoxide (The Hospital pharmacy, Capital Region of Denmark) and 98% PBS and mixed with 500 µL Montanide ISA-51 (Seppic Inc., Air Liquide Healthcare specialty ingredients, Paris, La Defence, France).
Clinical and toxicity assessment
Patients were eligible for response evaluation after 12 weeks of treatment. Clinical efficacy was assessed using positron emission tomography-computed tomography (PET-CT) scans 18F-deoxyglucose-PET/CT (18F-FDG-PET/CT) scans at baseline (up to 28 days before treatment initiation) and every third month thereafter until progression. CT scans were generally evaluated according to RECIST 1.1 (21).
All patients receiving one or more treatment courses were evaluable for toxicity. Toxicity was assessed using Common Terminology Criteria for Adverse Events (CTCAE version 3.0).
Blood samples
Peripheral blood mononuclear cells (PBMC) were purified from heparinized blood using lymphoprep™ (StemCell Technologies) density gradient centrifugation in LeucoSep™ tubes (Greiner Bio-One). After processing, cells were frozen in Nunc 1.8-mL Cryotube (Thermo Scientific) at a concentration of 10e6 cells/mL and stored at −140 °C in 90% human AB serum (HS) (Sigma Aldrich) and 10% dimethyl sulphoxide (DMSO) (Herlev Hospital Pharmacy). Serum was separated by centrifugation of full-blood collected in an 8-mL Vacuette® gel-tube containing clot activator (Greiner Bio-One), aliquoted in Nunc 1.8-mL Cryotube (Thermo Scientific) and stored at −80 °C until analysis. For PBMC, 100 mL heparinized blood were drawn per sampling and for serum 8 mL full blood were drawn per sampling. Blood samples were obtained at baseline, week 4, week 8 and week 12. Until progression, patient samples were drawn each month for the first 6 months and thereafter every third month.
Ex vivo enzyme-linked immunospot (ELISPOT)
ELISPOT analyses were performed in accordance with the Association for Cancer Immunotherapy immunoguiding program (CIP) guidelines (http://cimt.eu/cimt/files/dl/cip_guidelines.pdf). Vaccine responses were first assessed directly, ex vivo, and later indirectly, in vitro, see below. Cryopreserved PBMC were thawed in 37 °C RPMI medium 1640 + GlutaMAX (Life Technologies), washed twice in RPMI medium 1640 + GlutaMAX and resuspended in X-vivo 15 (Lonza Bioscience) containing 5% HS (Sigma Aldrich) and rested overnight in 24-well plates. Nitrocellulose bottomed 96-well plates were coated overnight with primary monoclonal antibodies (Mab) against human gamma interferon (IFN-γ) clone 1-D1K (Mabtech). The wells were washed in PBS (Sigma Aldrich) and blocked with X-vivo 15 media for 2 hours in 37 °C humidified atmosphere supplemented with 5% CO2. Cells were added to wells in 100 µL at concentrations of 5e6 cells/mL and 1.5e6 cells/mL along with 100 µL of 0.01 mM peptides in a X-vivo dilution. Peptides used were IDO5 (KJ Ross-Petersen APS, Klampenborg, Denmark), Sur1M2 (KJ Ross-Petersen APS, Klampenborg, Denmark), a negative control, C20, an HIV-derived epitope, amino acid one-letter code ILKEPVHGV (KJ Ross-Petersen APS, Klampenborg, Denmark) and a pool of human cytomegalovirus, Epstein-Barr virus and influenza virus epitopes (Mabtech) were used as a positive control (22). The plates incubated overnight at 37 °C in a humidified atmosphere supplemented with 5% CO2. Medium was discarded the following day and after washing in PBS, biotinylated anti-human IFN-γ, 7-b6-1 Mab (Mabtech) was added and plates were incubated 2 hours at room temperature, before washing with PBS and subsequently adding streptavidin alkaline phosphatase (Mabtech). Plates were incubated 1 hour at room temperature then washed in PBS before BCIP/NBT substrate system (Mabtech) was added to induce a color reaction. Reaction was terminated at the emergence of dark purple spot by washing thoroughly with de-mineralized water. The spots were counted using the ImmunoSpotSeries 2.0 Analyzer (CTL Analyzers). ELISPOT assay was interpreted using the method of distribution free resampling (DFR) as described by Moodie et al. (23). Experiments were done in triplicates and results are presented as the mean number of spots in C20 stimulated wells subtracted.
In vitro ELISPOT
After thawing, as described above, PBMC were resuspended in X-vivo 15 and seeded in a 24-well plate with 4×106 cells per well. The cells were pulsed with IDO5 for 1 hour at room temperature and then Sur1M2 for 1 hour at room temperature both at a concentration of 5 µg/mL. This was done because of Sur1M2’s relative higher affinity than IDO5. After pulsing, 1.5 mL of X-vivo 15 with 5% HS were added to the cells and the plates incubated overnight at 37 °C in a humidified atmosphere supplemented with 5% CO2. On day 1, 240 international units (IU) of IL-2 (Novartis, Denmark) were added to each well. After 7 days, the cells were washed in X-vivo 15 and used in an ELISPOT assay as above. During incubation, the condition of the cells and the color of the media were regularly checked and new media was applied when needed.
Enrichment for antigen specific T-cells
PBMC were pulsed with IDO5 and Sur1M2 in X-vivo 15 in a 24-well plate for 2 hours at room temperature at a concentration of 20 µM and 3–4×106 cells per well. Then 1.5 mL of X-vivo 15 with 5% HS were added and the cells incubated at 37 °C in a humidified atmosphere supplemented with 5% CO overnight. The day after peptide stimulation, 240 IU of IL-2 (Novartis, Denmark) were added to the wells. The cells were in culture for 3 weeks and were re-stimulated with peptide in log10 decreasing concentration of peptide each week. The cells were checked every day and new media was added every 10 days or when needed, assessed by cell number and color of the media. After 3 weeks, the cells were sorted using the TNF-α secretion assay (Miltenyi Biotec) according to the manufacturer’s instructions and expanded in a rapid expansion protocol (REP), see below. Prior to sorting, the cells were re-stimulated with 20 µM peptide.
REP
T-cells enriched for IDO/Sur1M2-reactivity were expanded, and approximately 1–2×105 cells were used initially. Cultures were initiated in upright T25 flasks (Nunc) containing 20 mL X-vivo 15 (Lonza Bioscience) supplemented with 10% HS, 0.6 µg anti-CD3 (OKT3, Janssen-Cilag), 6,000 IU/mL IL2 (Proleukin, Novartis), 1.25 µg/mL Fungizone (Squibb), 100+100 IU/mL penicillin-streptomycin (Gibco, Life Technologies) and 2×107 feeder-cells. As feeder cells, allogeneic PBMC mixed from three donors were used. The feeder cells were thawed separately from each donor, washed in RPMI-1640 (Gibco, Life Technologies), γ-irradiated with 30 Gy and mixed together. The feeder cells were mixed with anti-CD3 and added to the T-cells with the rest of the media components. The media was changed after 5 days by carefully removing 10 mL media from the flasks and adding 10 mL of fresh media. Media was changed when needed assessed by cell number and media color. The cells were first moved to a T80 (Nunc) and later to a T175 (Nunc) as the number of cells increased. After 14 days, cells were harvested and analyzed for IDO/Sur reactivity or cryopreserved in 90% HS with 10% DMSO.
Intracellular cytokine staining (ICS)
ICS was performed in 96-well plates (Nunc, Fischer Scientific) with 5×105 cells/mL in X-vivo 15. PBMC were stimulated with 5 µg/mL of peptide in the presence of 1 µL/mL GolgiPlugTM (BD Biosciences). After 5 hours, cells were stained for surface antigens and dead cell markers with the following reagents: anti-CD3-PerCP, anti-CD8-FITC and LIVE/DEAD Fixable Near-IR Dead Cell Stain 7 (Life Technologies). Cells were fixated and permeabilized with BD Cytofix/Cytoperm kit (BD Biosciences) according to manufacturer’s instructions. After permeabilization, cells were stained for intracellular antigens with the following reagents: anti-IL2-PE, anti-IFNγ-APC, anti-TNFα-PE-Cy7 and anti-CD4-HV500. CD4 staining was done intracellular as stimulation of T-cells can induce internalization of CD4 protein (24). A minimum of 200,000 cells were acquired with the FACSCanto II (BD Biosciences) using FACSDiva software version 6.1.3 (BD Biosciences). FlowJo software version 10 (Tree Star, Ashland, USA) was used to analyze the data.
Flowcytometric analysis
PBMC samples were thawed in 37 °C RPMI medium 1640 + GlutaMAX (Life Technologies). Samples were washed in RPMI and stained in PBS (Lonza Bioscience) containing 0.5% bovine serum albumin. Regulatory T-cells cells were stained with the following antibodies: CD127-FITC, FoxP3-PE or IG2a-PE (purchased from BD Biosciences), CD4-APC (purchased from BD Biosciences), CD25-BV421 (purchased from Biolegend) and CD3-HV500 (purchased from BD Horizon). For intracellular staining of transcription factor FoxP3, we used Foxp3/Transcription Factor Staining Buffer set (eBioscience) according to guidelines issued by the manufacturer. For phenotyping of CD3+ T-cells, the following antibodies were used: CD45RA-FITC, CCR7-PE-Cy7, CD4-APC (purchased from BD biosciences), CD27-PerCP, CD8-BV421 (purchased from Biolegend) and CD3-HV500 (purchased from BD Horizon). To evaluate myeloid-derived suppressor cells (MDSCs), the following antibodies were used: CD33-FITC, CD124-PE, CD11b-APC (purchased from BD Pharmigen), HLA-DR-PerCP, lineage markers in the PE-Cy7 channel (CD19, CD3, CD56), CD14-BV421 (purchased from BD Biolegend) and CD15-HV500 (purchased from BD Horizon). Cells were acquired on a FACSCanto II using FACSDiva Software version 6.1.3 (BD Biosciences). Analysis of flow data was done using FlowJo software version 10 OSX (Tree Star, Inc., Ashland, USA). Phenotype data was additionally analyzed using SPICE (Bioinformatics and Computational Biosciences Branch, Rockville, USA).
Statistical analysis
Statistical calculations were performed using either GraphPad Prism (GraphPad Software, La Jolla, CA, USA) or R (a language and environment for statistical computing (R Core Team, Vienna, Austria). Groups were evaluated in intention-to-treat analysis. All tests were performed two-sided with P values below 0.05 considered significant.
Results
Demography
In total, 41 patients were screened for HLA-A2 tissue type in the period from February 2012 to August 2015; 17 patients were HLA-A2+ and assigned to the vaccine group. Patient characteristics are presented in Table 1. A total of 13 patients (73.5%) were staged as having M1c disease according to American Join Committee on Cancer (AJCC) grading system for malignant melanoma. Screening for brain metastasis was not performed routinely at inclusion, but 8 patients (47%) had confirmed brain metastasis upon inclusion.
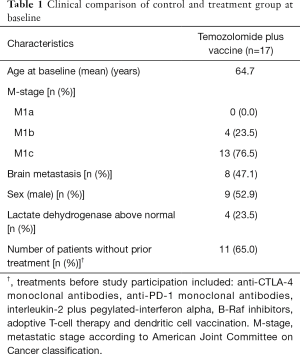
Full table
Clinical beneficial rates and survival estimates
Patients were treated for a median of 56 days (range: 28–70 days) and received a median of 6 (range: 2–8) vaccines. CBR was defined as patients having CR, PR or SD for a minimum of 6 months after treatment initiation evaluated according to RECIST (Figure 1A). According to this definition, 3 patients (18%) experienced clinical benefit, 1 patient had a confirmed PR and 2 patients showed SD as depicted in the spider plot (Figure 1B). Additionally, three patients demonstrated SD lasting less than 6 months. Imaging examples of tumor regression are shown in Figure S1.
As depicted in Figure 1C, we have observed a median survival of 35.4 weeks.
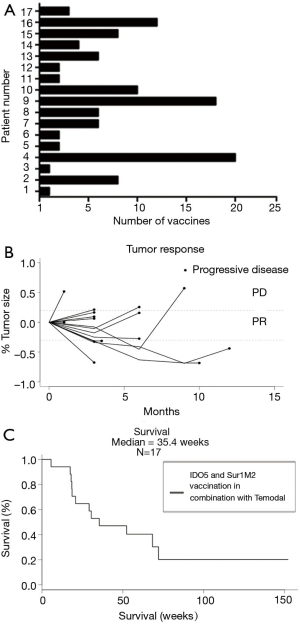
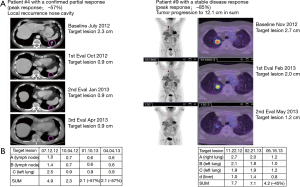
Four patients had a survival above 150 weeks. The only similarity among these four patients was treatment with an immune checkpoint inhibitor either prior to or post the vaccination trial. As recent publications have shown significant effect on survival when treating with immune checkpoint inhibitors, this could be the cause of their prolonged survival and it highlights the heterogeneity of the patient group.
Toxicity
Toxicity was scored in vaccinated patients. No grade 4 toxicity was observed as shown in Table 2. Grade 3 CTCAE toxicities were fatigue (15%), lymphopenia (15%), and leucopenia (8%), which are commonly observed during treatment with TMZ and leading to dose reduction. Nausea was observed in relation to brain metastases. Vaccine injection was associated with mild local reactions in the majority of patients due to the usage of two adjuvants. One patient developed arterial thrombosis in the left leg eventually leading to amputation. The event was assessed as unrelated to the temozolomide or vaccination. No severe local or systemic adverse events related to the vaccine were observed.
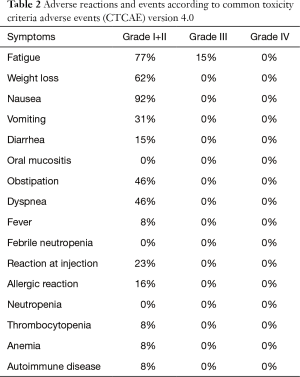
Full table
T-cell reactivity towards vaccine peptides
It has previously been shown that vaccination with the peptides IDO5 and Sur1M2 can induce expansion of peptide specific T-cells (12,19). In addition, for Sur1M2 the reactivity was associated with a longer overall survival. In this study, we did an initial screening for IFN-γ producing peptide specific T-cells with ex vivo IFN-γ ELISPOT in blood samples from five patients, reactivity was only demonstrable in three patients. Therefore, on the remaining patients, we performed in vitro IFN-γ ELISPOT to increase the sensitivity of the assay. For 12 of 17 patients, sufficient PMSCs material were available. Of these 12 patients, 9 patients remained in the study for 1st clinical evaluation point after 3 months. In total, we were able to demonstrate reactivity against IDO5 in 5 (42%) patients and reactivity against Sur1M2 in 5 (42%) patients of the 12 patients tested in both in vitro and ex vivo assays. Ex vivo ELISPOT responses are shown in Figure 2A for IDO5 and Figure 2B for Sur1M2 and in vitro ELISPIT responses are shown in Figure 2C,D. Of the 9 patients still included after 3 months, reactivity was demonstrated against Sur1M2 in 4 (44%) patients and against IDO5 in 3 (33%) patients.
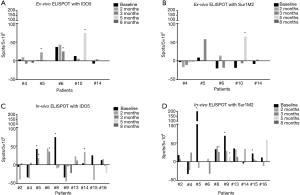
To further investigate the vaccine response, we established cultures specific for IDO5 and Sur1M2. Cells were cultured in the presences of a log10 decreasing stimulation with peptides once each week for 3 weeks. Afterwards, cells were stimulated with a high concentration of peptide and cells secreting TNF-α were positively sorted and expanded in a REP. We were able to establish a Sur1M2 specific culture with PBMC from patients #5 and #10, as depicted in Figure 3A,B. Patient #5’s culture demonstrated both Sur1M2 specific CD8+ and CD4+ T-cells, while patient #10’s culture demonstrated a major Sur1M2 CD4+ specific population (41.2%). Hence, the modified survivin-epitope Sur1M2, used in many preclinical and clinical studies, is not only restricted to HLA-A2 but is in addition able to induce survivin-specific CD4+ T-cells. This specific CD4 response in patient #10 was further investigated and we found that such T-cells were functionally relevant as they recognize cells with intracellular expression of survivin as published elsewhere (25). We tested if vaccine responses at any time point were correlated to a prolonged survival but we found no association, data not shown.
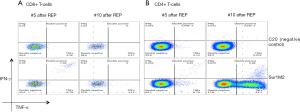
Lymphocyte counts were routinely obtained during therapy with TMZ and lymphopenia is linked to TMZ treatment. We assessed a possible correlation between TMZ-induced lymphopenia and vaccine responses by comparing lowest measured absolute lymphocyte count (ALC) in patients with or without a vaccine response and found a trend without statistical significance towards lower ALC in patients with detectable reactivity (P=0.085) as seen in Figure 4.
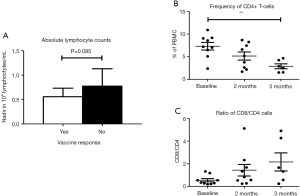
CD4+ reduction during treatment
TMZ has been shown to decrease the relative number of CD4+ T-cells, increase the relative number of CD8+ T-cells and induce a shift towards a relatively more differentiated phenotype of both CD4+ and CD8+ T-cells (3). To investigate the influence of adding a vaccine to TMZ treatment, we performed flow cytometry analysis on PBMC at baseline, after 2 months of treatment and after 3 months of treatment. PBMC were gated for lymphocytes, live cells and singlets as shown in Figure S2. Sufficient PBMC samples were available for analysis in 9 patients, of these patients, 6 were still included in the study after 3 months. During treatment, the proportion of CD3+ cells decreased non-significantly, CD4+ T-cells showed a significant reduction (P=0.01) after 3 months of treatment whereas CD8+ T-cells did not demonstrate any significant changes during treatment, as presented in Figure 5. To investigate the treatment-related dynamics of different phenotypes of CD4+ and CD8+, we assessed T-cell expression of CD27, CCR7 and CD45RA, as presented in Figure 5A,B. Though not significant, we observed a relative depletion in the CD27+CCR7+CD45RA+ cells (P=0.25 for CD4+ and P=0.08 for CD8+) and a relative induction of CD27−CCR7−CD45RA− cells (P=0.32 for CD4+ and P=0.08 for CD8+). CD27+CCR7+CD45RA+ cells are deemed as a naïve population, thus, during treatment there was a tendency towards decreasing frequencies of naïve CD4+ and CD8+ T-cells and increasing frequencies of memory CD4+ and CD8+ T-cells. This indicates, though without statistical significance, that the naïve subpopulation might be more sensitive to TMZ treatment and that a drop in naïve CD4+ T-cells is the subset mostly responsible for the decrease of absolute CD4+ T-cells.
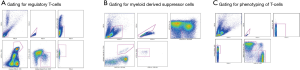
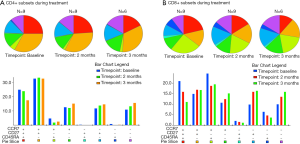
Changes in regulatory cells during treatment
The frequency of regulatory T cells (Tregs) and monocytic myeloid-derived suppressor cells (moMDSCs) were assessed for treatment-associated changes as depicted in Figure S3. Nine patients were available for testing; of these 6 were still included after 3 months. Gating strategy is also shown in supplementary material.
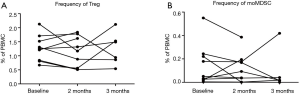
During treatment, 9 patients (53%) required treatment with glucocorticoids, either orally or intravenously, mainly due to symptomatic brain metastasis and they were therefore excluded from the trial. Eight additional vaccine patients (47%) discountinued treatment before first response evaluation due to rapid disease progression.
Tregs defined as PBMC CD3+CD4+CD25highCD127−FoxP3+ did not change significantly during treatment, but remained at a stable level of mean 1.0–1.5% of PBMCs. MoMDSC defined as PBMCs negative for lineage markers CD3, CD19 and CD56 and HLA-DR−/lowCD14+CD11+CD124−CD33+ decreased in some patients during treatment but not in general.
Clinical effect of vaccination compared to standard care
In an intention-to-treat manner, we compared the vaccinated HLA-A2+ patients and HLA-A2− patients receiving standard temozolomide therapy at baseline and found no significant differences between the two groups, though we did observe a non-significant tendency towards a lactate dehydrogenase (LDH) above normal value in the HLA-A2− group as seen in Table S1. Furthermore, we compared the CBR and survival as seen in Figure S4 and found no significant difference between the two groups.
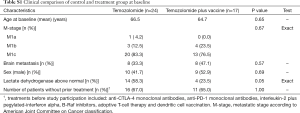
Full table
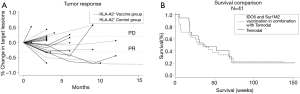
Discussion
Chemotherapy and vaccination have previously been regarded as conflicting treatment modalities. During the last decade, this hypothesis has been challenged by data showing that the immune modulating effects of chemotherapies can lead to increased immunity through e.g., inhibition of immune inhibitory cells (26). Hence, Dueland et al. treated metastatic melanoma patients with a peptide vaccine against telomerase in combination with TMZ (27) and reported an increased number of telomerase specific T-cell responses compared with other vaccine studies using the same peptides (28,29). In this present study, we investigated the clinical and immunological effect of treatment with an IDO5 and Sur1M2 peptide vaccine in combination with TMZ.
Our primary end point was CBR which was measured to 18%, including one patient with confirmed PR, in the vaccination group. At the end of our study, four patients were still alive, but not receiving treatment because of disease progression. The trial was prematurely terminated due to recruitment difficulties as TMZ treatment moved from 1st or 2nd line to 2nd or later line of treatment with the approval of ipilimumab (30). As a consequence, the majority of available patients were less likely to respond to study treatment due to poor performance status or need of prednisone treatment. To evaluate the immunological effect of the vaccine, we screened patient PBMC samples using ex vivo and in vitro IFN-γ ELISPOT assays and found responses against IDO5 in 5 (42%) of the patients tested with two responses being present at base line. Likewise, responses against Sur1m2 were detected in 5 patients (42%) with three responses being present at baseline. Previously spontaneous reactivity against IDO5 in PBMC from MM patients has been reported in 3/6 patients by in vitro ELISPOT assays by Sørensen et al. (11) and spontaneous reactivity against Sur1M2 in PBMC from MM was detected in 7/14 patients by in vitro ELISPOT assays by Andersen et al. (31). The baseline reactivity is lower in this study and this could have an impact on the ability of the vaccine to induce reactivity. For vaccine efficacy comparison, Iversen Iversen et al. (12) demonstrated vaccine specific T-cells in all treated patients when using IDO5 as vaccine monotherapy of metastatic lung cancer while Becker et al. (19) have reported vaccine specific T-cells in 13/28 patients using Sur1M2 for patients with MM. Stage M1c disease was found to correlate with impaired vaccine responses in that study, which could offer an explanation to the relatively low vaccine response level in our study as 76.5% of the patients had stage M1c disease. Iversen et al. (12) observed long-lasting disease stabilization for NSCLC patients with a IDO5 peptide reactivity prior to vaccination with IDO5. In our study, two patients had baseline reactivity against IDO5, but it was not associated with prolonged survival.
The moderate number of patients displaying vaccine specific immune responses could also be caused by TMZ-induced reduction in PBMC sample quantity and quality.
We further characterized vaccine reactive TNF-α secreting T cell in two patients by sorting and expansion and were able to demonstrate Sur1M2 reactive CD8+ T-cells producing both IFN-γ and TNF-α suggestive of CTLs (32). Furthermore, we detected a CD4+ T-cell population producing mostly TNF-α (25). The clinical effect of the CD4+ vaccine specific T-cells likely depends on the functional subtype. In MM CD4+ Treg and Th17 have been described to correlate to a poor prognosis (33,34). Th1 and Th2 CD4+ T-cells has, however, been described to possess anti-tumor effect either directly or indirectly by attraction or activation of other immune cells (35,36).
In a recent study (3), it was described that TMZ treatment induced a significant decrease in circulating CD4+ T-cells, a relative decrease of naïve T-cells and a relative increase in differentiated T-cells. These findings were confirmed in the present study but due to a smaller patient cohort the changes did not meet the level of statistical significance.
In the study by Iversen et al. (3), reactivity towards several known TAA were assessed before and after TMZ treatment. It showed that frequencies of CD8+ TAA specific T-cells either stabilized or increased after TMZ treatment and in some patients TMZ treatment even revealed TAA specific T-cells, which were not detectable before treatment. Here we assessed whether the lymphodepleting effects of TMZ could influence induction of vaccine specific responses and found a borderline significant association between reduced ALC and vaccine response.
As IDO expression can induce Tregs (9) and TMZ can reduce Treg levels (3), we hypothesized that vaccinating against IDO in combination with TMZ would have a synergistic effect on the Treg frequency in the blood. Such an effect would be interesting as it might contribute to an augmented vaccine efficacy and may affect disease prognosis (37). However, we observed no significant change in Treg frequencies during therapy. In some patients, we observed a fall in the frequency of moMDSC during treatment. The two patients, #5 and #10, with the largest relative fall in frequency of moMDSC also showed reactivity against IDO5. In another recent study from our group, patients were treated with Ipilimumab and a long peptide vaccine against IDO. Here, a significant fall in moMDSC frequency was observed (38).
In summary, we safely treated 17 patients with a Sur1M2 and IDO5 peptide vaccine in combination with TMZ. We were able to induce vaccine specific immunity in a significant number of patients; however, demonstration of clinical benefit was hampered by early and rapid disease progression including progressive brain metastases leading to need of high dose prednisone.
Acknowledgements
Funding: The project was partly funded by research grants from The Danish Cancer Society and the Danish Council for Independent Research for Medical Sciences.
Footnote
Conflicts of Interest: The authors have no conflicts of interest to declare.
Ethical Statement: The study was approved by Danish Health and Medicines Agency and the local Ethics Committee, Capital Region of Denmark (No. H-1-2011-136).
References
- Aranda F, Vacchelli E, Eggermont A, et al. Trial Watch: Peptide vaccines in cancer therapy. Oncoimmunology 2013;2:e26621. [Crossref] [PubMed]
- Lake RA, Robinson BW. Immunotherapy and chemotherapy--a practical partnership. Nat Rev Cancer 2005;5:397-405. [Crossref] [PubMed]
- Iversen TZ, Brimnes MK, Nikolajsen K, et al. Depletion of T lymphocytes is correlated with response to temozolomide in melanoma patients. Oncoimmunology 2013;2:e23288. [Crossref] [PubMed]
- Kaufmann R, Spieth K, Leiter U, et al. Temozolomide in combination with interferon-alfa versus temozolomide alone in patients with advanced metastatic melanoma: a randomized, phase III, multicenter study from the Dermatologic Cooperative Oncology Group. J Clin Oncol 2005;23:9001-7. [Crossref] [PubMed]
- Tarhini AA, Kirkwood JM, Gooding WE, et al. A phase 2 trial of sequential temozolomide chemotherapy followed by high-dose interleukin 2 immunotherapy for metastatic melanoma. Cancer 2008;113:1632-40. [Crossref] [PubMed]
- Kessler JH, Mommaas B, Mutis T, et al. Competition-based cellular peptide binding assays for 13 prevalent HLA class I alleles using fluorescein-labeled synthetic peptides. Hum Immunol 2003;64:245-55. [Crossref] [PubMed]
- Uyttenhove C, Pilotte L, Théate I, et al. Evidence for a tumoral immune resistance mechanism based on tryptophan degradation by indoleamine 2,3-dioxygenase. Nat Med 2003;9:1269-74. [Crossref] [PubMed]
- Munn DH, Sharma MD, Baban B, et al. GCN2 kinase in T cells mediates proliferative arrest and anergy induction in response to indoleamine 2,3-dioxygenase. Immunity 2005;22:633-42. [Crossref] [PubMed]
- Baban B, Chandler PR, Sharma MD, et al. IDO activates regulatory T cells and blocks their conversion into Th17-like T cells. J Immunol 2009;183:2475-83. [Crossref] [PubMed]
- Munir S, Larsen SK, Iversen TZ, et al. Natural CD4+ T-cell responses against indoleamine 2,3-dioxygenase. PLoS One 2012;7:e34568. [Crossref] [PubMed]
- Sørensen RB, Berge-Hansen L, Junker N, et al. The immune system strikes back: cellular immune responses against indoleamine 2,3-dioxygenase. PLoS One 2009;4:e6910. [Crossref] [PubMed]
- Iversen TZ, Engell-Noerregaard L, Ellebaek E, et al. Long-lasting disease stabilization in the absence of toxicity in metastatic lung cancer patients vaccinated with an epitope derived from indoleamine 2,3 dioxygenase. Clin Cancer Res 2014;20:221-32. [Crossref] [PubMed]
- Ambrosini G, Adida C, Altieri DC. A novel anti-apoptosis gene, survivin, expressed in cancer and lymphoma. Nat Med 1997;3:917-21. [Crossref] [PubMed]
- Grossman D, McNiff JM, Li F, et al. Expression and targeting of the apoptosis inhibitor, survivin, in human melanoma. J Invest Dermatol 1999;113:1076-81. [Crossref] [PubMed]
- Kawasaki H, Altieri DC, Lu CD, et al. Inhibition of apoptosis by survivin predicts shorter survival rates in colorectal cancer. Cancer Res 1998;58:5071-4. [PubMed]
- Islam A, Kageyama H, Takada N, et al. High expression of Survivin, mapped to 17q25, is significantly associated with poor prognostic factors and promotes cell survival in human neuroblastoma. Oncogene 2000;19:617-23. [Crossref] [PubMed]
- Altieri DC. Validating survivin as a cancer therapeutic target. Nat Rev Cancer 2003;3:46-54. [Crossref] [PubMed]
- Andersen MH, Pedersen LO, Becker JC, et al. Identification of a cytotoxic T lymphocyte response to the apoptosis inhibitor protein survivin in cancer patients. Cancer Res 2001;61:869-72. [PubMed]
- Becker JC, Andersen MH, Hofmeister-Müller V, et al. Survivin-specific T-cell reactivity correlates with tumor response and patient survival: a phase-II peptide vaccination trial in metastatic melanoma. Cancer Immunol Immunother 2012;61:2091-103. [Crossref] [PubMed]
- Oken MM, Creech RH, Tormey DC, et al. Toxicity and response criteria of the Eastern Cooperative Oncology Group. Am J Clin Oncol 1982;5:649-55. [Crossref] [PubMed]
- Watanabe H, Okada M, Kaji Y, et al. New response evaluation criteria in solid tumours-revised RECIST guideline (version 1.1). Gan To Kagaku Ryoho 2009;36:2495-501. [PubMed]
- Currier JR, Kuta EG, Turk E, et al. A panel of MHC class I restricted viral peptides for use as a quality control for vaccine trial ELISPOT assays. J Immunol Methods 2002;260:157-72. [Crossref] [PubMed]
- Moodie Z, Price L, Gouttefangeas C, et al. Response definition criteria for ELISPOT assays revisited. Cancer Immunol Immunother 2010;59:1489-501. [Crossref] [PubMed]
- Kemp K, Bruunsgaard H. Identification of IFN-gamma-producing CD4+ T cells following PMA stimulation. J Interferon Cytokine Res 2001;21:503-6. [Crossref] [PubMed]
- Nitschke NJ, Bjoern J, Met O, et al. Therapeutic Vaccination against A Modified Minimal Survivin Epitope Induces Functional CD4 T Cells That Recognize Survivin-Expressing Cells. Scand J Immunol 2016;84:191-3. [Crossref] [PubMed]
- Kershaw MH, Devaud C, John LB, et al. Enhancing immunotherapy using chemotherapy and radiation to modify the tumor microenvironment. Oncoimmunology 2013;2:e25962. [Crossref] [PubMed]
- Kyte JA, Gaudernack G, Dueland S, et al. Telomerase peptide vaccination combined with temozolomide: a clinical trial in stage IV melanoma patients. Clin Cancer Res 2011;17:4568-80. [Crossref] [PubMed]
- Hunger RE, Kernland Lang K, Markowski CJ, et al. Vaccination of patients with cutaneous melanoma with telomerase-specific peptides. Cancer Immunol Immunother 2011;60:1553-64. [Crossref] [PubMed]
- Brunsvig PF, Aamdal S, Gjertsen MK, et al. Telomerase peptide vaccination: a phase I/II study in patients with non-small cell lung cancer. Cancer Immunol Immunother 2006;55:1553-64. [Crossref] [PubMed]
- Hodi FS, O'Day SJ, McDermott DF, et al. Improved survival with ipilimumab in patients with metastatic melanoma. N Engl J Med 2010;363:711-23. [Crossref] [PubMed]
- Andersen MH, Pedersen LO, Capeller B, et al. Spontaneous cytotoxic T-cell responses against survivin-derived MHC class I-restricted T-cell epitopes in situ as well as ex vivo in cancer patients. Cancer Res 2001;61:5964-8. [PubMed]
- Andersen MH, Schrama D, Thor Straten P, et al. Cytotoxic T cells. J Invest Dermatol 2006;126:32-41. [Crossref] [PubMed]
- Ascierto PA, Napolitano M, Celentano E, et al. Regulatory T cell frequency in patients with melanoma with different disease stage and course, and modulating effects of high-dose interferon-alpha 2b treatment. J Transl Med 2010;8:76. [Crossref] [PubMed]
- Zou W, Restifo NP. T. (H)17 cells in tumour immunity and immunotherapy. Nat Rev Immunol 2010;10:248-56. [Crossref] [PubMed]
- Nishimura T, Iwakabe K, Sekimoto M, et al. Distinct role of antigen-specific T helper type 1 (Th1) and Th2 cells in tumor eradication in vivo. J Exp Med 1999;190:617-27. [Crossref] [PubMed]
- Eftimie R, Bramson JL, Earn DJ. Modeling anti-tumor Th1 and Th2 immunity in the rejection of melanoma. J Theor Biol 2010;265:467-80. [Crossref] [PubMed]
- Knol AC, Nguyen JM, Quéreux G, et al. Prognostic value of tumor-infiltrating Foxp3+ T-cell subpopulations in metastatic melanoma. Exp Dermatol 2011;20:430-4. [Crossref] [PubMed]
- Bjoern J, Iversen TZ, Nitschke NJ, et al. Safety, immune and clinical responses in metastatic melanoma patients vaccinated with a long peptide derived from indoleamine 2,3-dioxygenase in combination with ipilimumab. Cytotherapy 2016;18:1043-55. [Crossref] [PubMed]
Cite this article as: Nitschke NJ, Bjoern J, Iversen TZ, Andersen MH, Svane IM. Indoleamine 2,3-dioxygenase and survivin peptide vaccine combined with temozolomide in metastatic melanoma. Stem Cell Investig 2017;4:77.