Vaccination with induced pluripotent stem cells confers protection against cancer
“… I’m just a kid again doing what I did again…”
The old lyric (from “When the Red, Red Robin Comes Bob, Bob, Bobbin’ Along”) might be used to describe the origins of many types of cancer. In this case, the kid might be a mutated stem cell and cancers arising from it may replay many events in embryogenesis including the formation of trophoblasts, the associated development of a dedicated (if somewhat chaotic) vasculature and migration (metastasis). Now it is becoming likely that early embryonic gene products—absent in adult organisms—might be useful for the production of broad spectrum prophylactic cancer vaccines.
Most research projects do not last more than a century. But this one has. More than one hundred years have passed since the first report by Schöne and colleagues showed that vaccination of animals with fetal material might prevent the outgrowth of tumors (1). There is abundant evidence that tumor cells and pluripotent stem cells share antigens (2). It now appears that most, if not all, types of neoplastic cells express certain embryonal antigens (2-5), a phenomenon earlier termed “retrogenetic expression” (3). Immune cells (specifically T cells) can be primed to mount anti-cancer attack by these embryonic antigens (also called carcinoembryonic antigens). This is because many embryonic gene products are not expressed in adult organisms and are not included in the repertoire of “self” which is set by thymic selection near the end of gestation. Such “non-self” gene products are, pari passu, immunogenic.
Exploiting such embryonic antigen immunogenicity, Kooreman et al. have designed a unique stem cell-based vaccine that could stimulate the immune system to recognize shared oncofetal antigens and confer protection against tumors. In their study described in Cell Stem Cell (6), Kooreman and colleagues use syngeneic irradiated induced pluripotent stem cells (iPSCs) in a prophylactic setting to vaccinate against “other” non-mutated neo-antigens, i.e., tumor antigens derived from proteins that are only expressed during embryonic development and not in adult tissues (6).
For a cancer vaccine to be successful, the vaccine must be effectively processed by antigen-presenting cells (APCs) and transported to draining lymph nodes (dLN), where the presented tumor-associated antigens (TAAs) activate tumor-specific cytotoxic CD8+ T cells. While most immunotherapeutic vaccines for solid tumors have involved promotion of immune responses against individual TAAs, these have generally been ineffective. Nonetheless, if a source of multiple tumor associated neo-antigens presented by a variety of tumors (but not by adult tissues) were available, the probability of creating a broad spectrum cancer vaccine would be vastly improved. Based on this rationale, Kooreman and colleagues have designed their whole cell vaccine consisting of multiple tumor-derived antigens in order to induce T cell-mediated immune responses against various cancer cells.
Using RNA sequencing, the authors show that both human and mouse iPSCs share expression of several different genes with embryonal stem cells (ESCs) and cancer tissues but not with healthy tissues, revealing important antigenic overlap between pluripotent stem cells and cancer cells of varied origin (6). Studies from our lab and from several other groups support this theory of antigenic similarity between stem cells and cancer cells. Leukemia stem cells in acute myeloid leukemia have been shown to be maintained in a self-renewing state by an enriched transcriptional program that is also expressed in embryonic stem cells (4). These results were recapitulated in human breast cancer when Weinberg’s group reported that poorly differentiated, highly aggressive human breast cancers contain subpopulations of cells that have embryonic stem cell gene expression signatures. This gene signature was most prevalent in estrogen receptor negative breast cancer patients who generally fail to respond to hormonal therapies used for treating breast cancer (5). Recent studies by Tuohy’s group have shown that immunotherapeutic targeting of certain “retired antigens” can be effective in prevention of ovarian and breast cancers (7,8). Examples of such antigens include the extracellular domain of anti-Mullerian hormone receptor II (AMHR2-ED) and α-lactalbumin proteins; both of these are overexpressed in cancer cells but show limited expression in normal tissues and hence are attractive targets for prophylactic cancer vaccine with minimal risk for inducing toxicity (7,8).
In an earlier work (9), we vaccinated mice with irradiated allogeneic murine embryonic stem cells along with granulocyte-macrophage colony stimulating factor (GM-CSF) as an immunostimulatory adjuvant. We discovered that our whole-cell vaccination was 70–100% effective in preventing both implantable and carcinogen-induced lung adenocarcinomas (9). Along similar lines, Li et al. and Dong et al. have demonstrated that vaccination with either xenogeneic or syngeneic embryonic stem cells provides modest protection against tumors in mouse models of cancer (10,11). In these studies (including ours), the identity of cross-reactive antigens that are shared between tumors and embryonic stem cells remains unresolved; one plausible mechanism is that such allogeneic or xenogeneic vaccination will prime T cell responses that are likely to cross-react with multiple TAAs. The use of live embryonic stem cells—although irradiated—raises ethical concerns as well as the potential risks of inducing teratomas and autoimmunity. Kooreman et al. solve this major hurdle by generating iPSCs from autologous tissues for vaccine development (12) which are also useful for autologous transplantation (13,14).
The authors used a vaccination strategy that consisted of four weekly injections of irradiated syngeneic iPSCs in conjunction with the immunostimulatory CpG oligodeoxynucleotide (Toll-like receptor 9 agonist that elicits potent type I interferon responses) as an adjuvant (Figure 1). By immunizing mice with the iPSC vaccine either in a prophylactic or in an adjuvant tumor setting, robust anti-tumor responses (Figure 1) were obtained in transplantable and in clinically relevant orthotopic mouse cancer models of breast cancer, melanoma and mesothelioma. Importantly, iPSC-vaccinated mice remained tumor-free for over a year indicating that the vaccine confers long-term protection. This is an important observation because prior stem cell vaccine have generally used allogeneic stem cells. Given the near-identical nature of syngeneic iPSCs this suggests that the effective iPSC neoantigens are “baked in” to the normal genome.
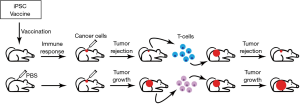
Kooreman et al. observed that iPSC vaccine-induced antibodies were reactive to iPSCs as well as to tumor cells and, to a minimal extent, normal fibroblasts. Vaccination also induced CD4+ (T helper) and CD8+ cytotoxic T cell responses against tumor cells in vitro suggesting ongoing anti-tumor immune response. In vivo, vaccination produced effective anti-tumor immune responses by upregulation of APCs in the dLNs that were accompanied by a systemic increase in CD4+ T helper and CD8+ cytotoxic T cell responses. Such a robust expansion of systemic T cell-mediated effector responses led to favorable increases in T effector/T regulatory cell ratio that is critical for rejecting tumors with highly immune-suppressive microenvironments such as melanoma (15). Consistent with this, the iPSC vaccine was not effective in reducing tumor growth in mice bearing established melanoma. This potential draw back of the vaccine can be overcome by combining the iPSC vaccine with other immunotherapeutic agents such as the immune checkpoint inhibitors (ICIs) that are currently in use for the treatment of patients with advanced melanoma (16,17). However, the Kooreman study conclusively shows that, in an adjuvant setting, after surgical resection of the primary melanoma, the iPSC vaccine was effective in rejecting remnant melanoma cells via activation of B cells and myeloid cells expressing inflammatory tumor-rejecting phenotypes and suppression of tumor-promoting type 17 cells (18). When mice received surgery but were left with microscopic tumors in dLNs, vaccination with either iPSC + CpG vaccine or CpG alone at the resection site could reduce tumor recurrence. However, when macroscopic tumors were left behind, only the iPSC + CpG combination vaccine reduced tumor regrowth in most mice (albeit this regimen was not 100% effective in reducing tumor recurrence). These critical experiments provide support for extending the use of iPSC vaccines as an adjuvant immunotherapeutic agent for patients whose primary tumor has been surgically removed leaving behind an “inflamed” tumor site.
Furthermore, Kooreman and colleagues show, with adoptive transfer studies, the transfer of primed T cells from iPSC-vaccinated mice into tumor-bearing mice results in tumor rejection in the recipient mice within 1 week of T cell transfer (Figure 1), which provides definitive proof that the vaccine-induced effector T cells are mediators of the observed tumor protection. Additionally, transfer of T cells from iPSC-vaccinated tumor-bearing mice into immuno-deficient recipient mice that were injected with iPSCs resulted in a reduction in teratoma size in the recipients. These data strongly suggest that the anti-tumor immunity generated by the iPSC vaccine is a result of shared epitopes between iPSCs and cancer cells and that the vaccine-induced T cell-mediated effector responses are cross-reactive to these shared epitopes.
Immunotherapy with ICIs, such as pembrolizumab (anti-PD-1 antibody) and ipilimumab (anti-CTLA-4 antibody), which unleash a patient’s own T cells to kill tumors, has emerged as a powerful therapeutic strategy for multiple cancer types (19,20). In patients responding to ICI therapy, neoantigen-specific CD8+ T cell responses parallel tumor regression, suggesting that ICI therapy enhances neoantigen-specific T cell reactivity (21). One of the best-described predictive biomarkers of immunotherapeutic responsiveness is tumor somatic mutation rates which dictate neoantigen load (22) and subsequent CD8+ T cell responses (23,24). Current clinical data indicate that immunotherapeutic efficacy is generally restricted to cancer types with higher mutational load (21) and abundant neoantigens (“hot” tumors; e.g., melanoma, lung cancer). In this context, it remains to be evaluated how the iPSC vaccine efficacy (especially in patients harboring hot tumors) will relate to overall embryonic antigen expression.
Immunotherapy is generally thought to be less toxic than other cancer treatment modalities (e.g., chemotherapy) and autoimmune responses to immunotherapy such as vitiligo in melanoma patients often adumbrate effective anti-tumor responses. However, autoimmunity and immune-related adverse events (irAEs) may be an Achilles heel of immunotherapy. It is therefore comforting that Kooreman et al. find that iPSC vaccination in syngeneic mouse tumor models is well tolerated as judged by organ histology, absence of anti-nuclear antibodies, and normal animal weight gain. Furthermore, the authors demonstrate that iPSC vaccination results in lower circulating levels of multiple cytokines (IL-6, IL-12, and IFN-γ) known to mediate therapeutic toxicity (e.g., IL-6- and IL-12-mediated cytokine release syndrome observed in patients receiving CAR-T cell therapies). Although the preclinical data observed with iPSC vaccine are extremely encouraging, laboratory mouse studies are poor predictors of therapeutic irAEs due to heterogeneity in genetic backgrounds and/or inflammatory status in laboratory maintained mice, and caution should be used when translating the whole cell iPSC vaccine strategy to the clinic.
The vaccination approach described by Kooreman and colleagues motivate further questions: (I) How effective is this vaccination in poorly immunogenic tumors that display a significant loss of tumor cell class I expression? (II) Do the antibodies generated by the vaccine directly bind tumor cells and initiate antibody-dependent cellular cytotoxicity (ADCC)? (III) Does the iPSC vaccine stimulate the innate arms (NK- mediated killing) of tumor immunity? (IV) Is the vaccine effective in spontaneous mouse models of cancers? (V) What is the nature of the (presumably multiple) antigens which lead to anti-tumor T effector responses? The authors suggest several different target antigenic candidates but the exact identity of the predominant, shared epitopes will need to be defined in the future using multiplex assay platforms. Such identification will also aid in developing TAA-derived vaccines that are personalized for individual cancer patients (similar to the personalized vaccines currently being developed for patients’ mutated neoantigens) as well as overcoming risk-factors associated with using whole-cell vaccines (teratomas/autoimmunity). Personalized immunotherapy treatments that involve reprogramming of patients’ own cells prove to be very expensive with complex manufacturing and storage logistics (e.g., the FDA-approved PROVENGE vaccine for prostate cancer treatment). Although iPSCs can be engineered as an allogeneic vaccine consisting of multiple TAAs, it will be imperative to estimate the production costs of such an “off-the-self” iPSC-based vaccination strategy.
The next step will be to conduct preclinical studies and test the efficacy of the iPSC vaccine in combination with other immunotherapies such as ICIs or other immune adjuvants (e.g., CD40 monoclonal antibodies and immunostimulatory cytokines). Such combinatorial strategies are likely to potentiate the anti-tumor efficacy of the vaccine, aid in overcoming the tolerance induced by tumor microenvironment and, thus, be beneficial to patients with even advanced cancers.
In summary, Kooreman et al. have described a novel immunotherapeutic vaccination strategy with iPSCs that is very effective in preventing the outgrowth of a variety of tumors in preclinical mouse models. Importantly, anti-tumor efficacy of the iPSC-based vaccine was associated with robust CD8+ T effector responses with no detectable toxicity. Further optimization and translation of this unique iPSC vaccine-based treatment strategy will greatly increase the chances of its success in the clinic and for attaining durable therapeutic responses in cancer patients.
If it proves possible to produce a similar vaccine for cancer prophylaxis and therapy then we may “… cheer up… live love laugh and be happy…” (back to the Red Robin).
Acknowledgements
Funding: This work was supported by grants from National Institutes of Health (CA198249 to K Yaddanapudi, AA018016-01 to JW Eaton, CA106599 and CA175003 to C Li), Free to Breathe Research Grant (to K Yaddanapudi), and Commonwealth of Kentucky Research Challenge Trust Fund (to JW Eaton).
Footnote
Conflicts of Interest: The authors have no conflicts of interest to declare.
References
- Schöne G. Untersuchungen über Karzinomimmunität bei Mäusen. Münchener Medizinische Wochenschrift 1906;51:2517-9.
- Brewer BG, Mitchell RA, Harandi A, et al. Embryonic vaccines against cancer: an early history. Exp Mol Pathol 2009;86:192-7. [Crossref] [PubMed]
- Stonehill EH, Bendich A. Retrogenetic expression: the reappearance of embryonal antigens in cancer cells. Nature 1970;228:370-2. [Crossref] [PubMed]
- Somervaille TC, Matheny CJ, Spencer GJ, et al. Hierarchical maintenance of MLL myeloid leukemia stem cells employs a transcriptional program shared with embryonic rather than adult stem cells. Cell Stem Cell 2009;4:129-40. [Crossref] [PubMed]
- Ben-Porath I, Thomson MW, Carey VJ, et al. An embryonic stem cell-like gene expression signature in poorly differentiated aggressive human tumors. Nat Genet 2008;40:499-507. [Crossref] [PubMed]
- Kooreman NG, Kim Y, de Almeida PE, et al. Autologous iPSC-Based Vaccines Elicit Anti-tumor Responses In Vivo. Cell Stem Cell 2018;22:501-13.e7. [Crossref] [PubMed]
- Mazumder S, Johnson JM, Swank V, et al. Primary Immunoprevention of Epithelial Ovarian Carcinoma by Vaccination against the Extracellular Domain of Anti-Mullerian Hormone Receptor II. Cancer Prev Res (Phila) 2017;10:612-24. [Crossref] [PubMed]
- Jaini R, Kesaraju P, Johnson JM, et al. An autoimmune-mediated strategy for prophylactic breast cancer vaccination. Nat Med 2010;16:799-803. [Crossref] [PubMed]
- Yaddanapudi K, Mitchell RA, Putty K, et al. Vaccination with embryonic stem cells protects against lung cancer: is a broad-spectrum prophylactic vaccine against cancer possible? PLoS One 2012;7. [Crossref] [PubMed]
- Li Y, Zeng H, Xu RH, et al. Vaccination with human pluripotent stem cells generates a broad spectrum of immunological and clinical responses against colon cancer. Stem Cells 2009;27:3103-11. [PubMed]
- Dong W, Du J, Shen H, et al. Administration of embryonic stem cells generates effective antitumor immunity in mice with minor and heavy tumor load. Cancer Immunol Immunother 2010;59:1697-705. [Crossref] [PubMed]
- Takahashi K, Tanabe K, Ohnuki M, et al. Induction of pluripotent stem cells from adult human fibroblasts by defined factors. Cell 2007;131:861-72. [Crossref] [PubMed]
- Kooreman NG, Wu JC. Tumorigenicity of pluripotent stem cells: biological insights from molecular imaging. J R Soc Interface 2010;7 Suppl 6:S753-63. [Crossref] [PubMed]
- de Almeida PE, Meyer EH, Kooreman NG, et al. Transplanted terminally differentiated induced pluripotent stem cells are accepted by immune mechanisms similar to self-tolerance. Nat Commun 2014;5:3903. [Crossref] [PubMed]
- Zou W. Immunosuppressive networks in the tumour environment and their therapeutic relevance. Nat Rev Cancer 2005;5:263-74. [Crossref] [PubMed]
- Zappasodi R, Merghoub T, Wolchok JD. Emerging Concepts for Immune Checkpoint Blockade-Based Combination Therapies. Cancer Cell 2018;33:581-98. [Crossref] [PubMed]
- Overwijk WW. Cancer vaccines in the era of checkpoint blockade: the magic is in the adjuvant. Curr Opin Immunol 2017;47:103-9. [Crossref] [PubMed]
- He D, Li H, Yusuf N, et al. IL-17 promotes tumor development through the induction of tumor promoting microenvironments at tumor sites and myeloid-derived suppressor cells. J Immunol 2010;184:2281-8. [Crossref] [PubMed]
- Hodi FS, O'Day SJ, McDermott DF, et al. Improved survival with ipilimumab in patients with metastatic melanoma. N Engl J Med 2010;363:711-23. [Crossref] [PubMed]
- Topalian SL, Hodi FS, Brahmer JR, et al. Safety, activity, and immune correlates of anti-PD-1 antibody in cancer. N Engl J Med 2012;366:2443-54. [Crossref] [PubMed]
- Rizvi NA, Hellmann MD, Snyder A, et al. Cancer immunology. Mutational landscape determines sensitivity to PD-1 blockade in non-small cell lung cancer. Science 2015;348:124-8. [Crossref] [PubMed]
- Schumacher TN, Schreiber RD. Neoantigens in cancer immunotherapy. Science 2015;348:69-74. [Crossref] [PubMed]
- McGranahan N, Furness AJ, Rosenthal R, et al. Clonal neoantigens elicit T cell immunoreactivity and sensitivity to immune checkpoint blockade. Science 2016;351:1463-9. [Crossref] [PubMed]
- Gros A, Parkhurst MR, Tran E, et al. Prospective identification of neoantigen-specific lymphocytes in the peripheral blood of melanoma patients. Nat Med 2016;22:433-8. [Crossref] [PubMed]
Cite this article as: Yaddanapudi K, Li C, Eaton JW. Vaccination with induced pluripotent stem cells confers protection against cancer. Stem Cell Investig 2018;5:23.