The past, present, and future of CRM1/XPO1 inhibitors
Introduction
The regulation of protein transport across the nuclear membrane is essential for maintaining cellular homeostasis but this process is altered in tumor cells (1). While smaller molecules can passively diffuse through the nuclear pore complex (NPC), larger cargo molecules (>40 kDa) require active transport via transport receptors (2-5). These receptor proteins belong to the karyopherin beta family and are further classified into importins (for nuclear import), exportins (for nuclear export), and transportins (for both import and export) (4). Exportins are better studied as potential targets in tumorigenesis (6-8). The most important and best studied exportin is CRM1, which forms a ring-like structure consisting of 21 HEAT repeats. Cargo proteins displaying a leucine-rich nuclear export signal (LR-NES) bind to the hydrophobic groove formed by HEAT repeats 11 and 12 on the outer convex surface of CRM1 (9,10). A small GTPase Ran loaded with GTP then binds cooperatively with CRM1 to power the translocation of the CRM1-cargo-RanGTP complex through the NPC. Once the complex reaches the cytoplasm, it encounters RanBP1 to facilitate cargo release, as well as RanGAP1 to catalyze the hydrolysis of RanGTP to RanGDP (4,10). This process is tightly regulated by the GTP exchange factor, regulator of chromosome condensation 1 (RCC1), which is an exclusive nuclear protein responsible for reforming RanGTP from RanGDP. Its cytoplasmic counterpart is RanGAP1, and both ensure tight control of the import-export process through maintaining the Ran GDP:GTP gradient (11). It is through this nucleus-to-cytoplasm translocation of regulatory proteins that allows the cell to direct its proliferation or apoptosis pathways (6).
CRM1: first forgotten, then rediscovered
CRM1 is present in all eukaryotic cells and was initially identified as a chromosomal mutation in the yeast schizosaccharomyces pombe (12). Also known as exportin 1 or XPO1, it is responsible for the transport of over 200 proteins, which include many tumor suppressor proteins (TSP) and oncoproteins (11,13). In addition, CRM1 plays an essential role in mitosis through (I) binding and targeting key mitotic proteins to specific areas of the mitotic spindle; and (II) stabilizing the microtubule kinetochore to promote proper chromosomal segregation (14). Inhibition of RCC1, which regulates CRM1, by actinomycin D resulted in severe spindle assembly defects and mitotic catastrophe (15). Among the list of CRM1-mediated proteins are p53, FOXOs, p27, nucleophosmin, BCR-ABL, p21, PI3K/AKT, Wnt/β-catenin, NF-kB, APC, and Rb, all of which are significant targets in oncogenesis (6-8,11). Dysregulation of this transport process has been implicated in cancer, in addition to wound healing, inflammation, and viral infections (5,11). CRM1 overexpression has been demonstrated in both solid tumors and hematologic malignancies, and this overexpression is associated with a poorer prognosis and drug resistance (6,8). The exact mechanism by which this occurs remains unclear but is thought to involve altered transport mechanisms in favor of cytoplasmic localization and the modification of nuclear proteins to reveal their LR-NES enabling them to bind CRM1 (1,16).
Abnormal CRM1 upregulation can have several cancer-promoting consequences (6). Upregulation of CRM1 would allow more growth regulatory proteins, such as c-myc or BCR-ABL, to be transported into the cytoplasm and activate downstream signaling leading to sustained cell proliferation. Similarly, TSPs, such as Rb, p53, p21, or p27, are functionally inactivated upon export, hence removing the check on inappropriate cell growth. Similar disruptions would occur in the processes of apoptosis, DNA damage repair, chromosomal stabilization, and angiogenesis, just to name a few examples (6). Hence, inhibition of CRM1 activity became an attractive therapeutic target.
The first CRM1 inhibitor to be discovered was leptomycin B (LMB), which is naturally made by the bacteria Streptomyces (17,18). LMB was initially used as an anti-fungal agent, and its anti-cancer properties were discovered later (17,19). However, the clinical trials involving LMB were discontinued early due to profound cytotoxicity thought to be derived from permanent CRM1 inhibition (12,20). Several semi-synthetic CRM1 inhibitors were developed and studied in the pre-clinical setting, but unfortunately, none were ever entered into clinical trials (8). The next generation of compounds to be developed was collectively known as selective inhibitors of nuclear transport (SINE) compounds and include KPT-185, KPT-249, KPT-251, KPT-276, KPT-330, and KPT-335 (6). Similar to their predecessors, these molecules form covalent bonds to a cysteine residue (Cys528) on CRM1 (21). However, they improve upon the first-generation compounds by engaging in a slowly reversible covalent bonding, which improves upon the toxicity profile (22). The SINEs also cause a transient degradation of CRM1 that is reversible upon discontinuation of the SINE compound (6).
Somatic mutations in the CRM1 gene frequently occur in cancer cells, and they were initially identified in patients with CLL (23). Nearly 90% of the mutations involve an E571K amino acid change that resides near the NES-binding cleft. While this resulted in an increase in affinity for NES sequences bearing a more negatively charged C-terminal end, studies demonstrated that this mutational change did not impact the activity of CRM1 on nuclear transport or the efficacy of SINE compounds (24,25). However, if either a homozygous or heterozygous mutation involving the Cys528 residue was present, this conferred resistance to SINE compounds (26).
SINEs in the pre-clinical and clinical setting
Pre-clinical studies involving SINEs have demonstrated notable inhibition of tumor cell growth and promotion of cell apoptosis across a broad range of solid and hematologic malignancies, primarily through mediating the transport of key oncogenic proteins (see Table 1).
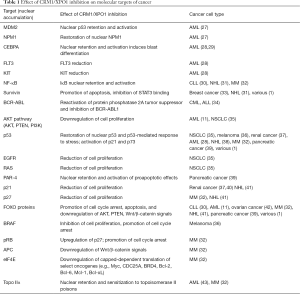
Full table
Selinexor or KPT-330 is the most well-known SINE and is currently undergoing study in about 60 clinical trials, involving lymphoma (i.e., Non-Hodgkin’s lymphoma, diffuse large B-cell lymphoma), sarcomas, lung cancer, gliomas, breast cancer, leukemia (ALL, AML, MDS), multiple myeloma (MM), gastric cancer, pancreatic cancer, esophageal cancer, prostate cancer, melanoma, colorectal cancer, thymic cancer, and gynecologic cancers (see Table 2) (44).
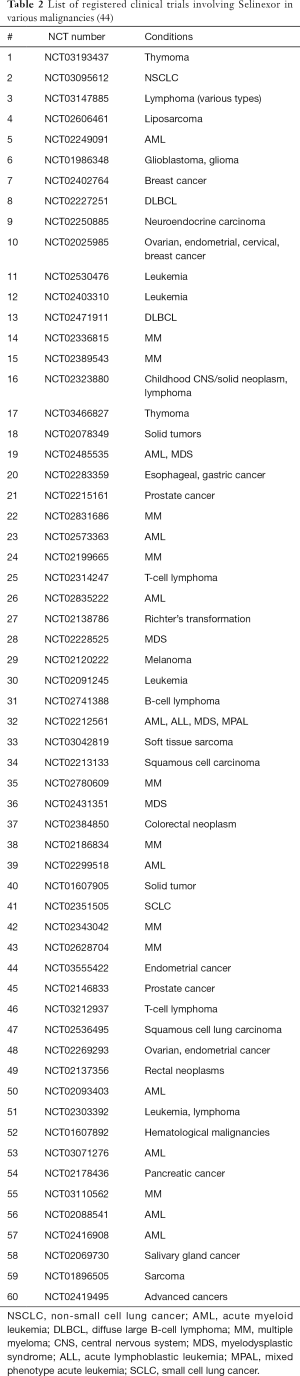
Full table
Lung cancer
Selinexor offers an attractive therapeutic strategy in non-small cell lung cancer (NSCLC) through demonstrating a dose-dependent growth inhibition and efficacy across multiple mutational variants in pre-clinical studies (35,45). Mutations in epidermal growth factor receptor (EGFR) and p53 have been associated with poorer outcomes (46,47). One study showed that selinexor was effective even in cells with mutations in EGFR, TP53, phosphatase and tensin homologue, RAS, and PIK3CA (35). Synergistic inhibition was also noted with the combination of selinexor and cisplatin (35). There is currently a multicenter phase I/II clinical trial of selinexor with docetaxel in previously treated KRAS-mutant NSCLC that is underway (48).
Breast cancer
Selinexor has shown promise in pre-clinical studies in triple-negative breast cancer by inducing cell apoptosis and reduced tumor growth (33,49). One study demonstrated that selinexor increased nuclear accumulation of the anti-apoptotic protein survivin and blocked STAT3 binding to the survivin promoter (33). The overall effect is decreased cytoplasmic levels of survivin and promotion of cell death. Currently, there are two phase II clinical trials of selinexor in patients with metastatic breast cancer.
Pancreatic cancer
The use of SINEs in pancreatic cancer remains largely in the pre-clinical setting. One study demonstrated that SINEs inhibited proliferation and promoted apoptosis of pancreatic cancer cells both in vitro and in vivo (39). Additionally, KPT-185 caused intranuclear accumulation of prostate apoptosis response-4 (PAR-4), which is a proapoptotic protein that is frequently downregulated in pancreatic cancer (39). KPT-330 in combination with gemcitabine demonstrated synergistic inhibition in pancreatic cancer cells in vitro (50).
Melanoma
Targeting BRAF and MEK pathways through kinase inhibition are important in the treatment of melanoma, but resistance to therapy eventually develops (8). SINE compounds induced cytostatic and pro-apoptotic effects in melanoma cell lines regardless of BRAF mutation status, as well as inhibition of tumor growth in vivo (36). The mechanisms of apoptosis appear to involve multiple cellular pathways involving inhibition of ERK phosphorylation, increased nuclear localization of p53 and phosphorylated MAPK, and G1/S phase cell cycle arrest (36,51). There is evidence of potential synergy between CRM1 and BRAF inhibition in BRAF-mutant melanoma (52), but this has yet to be tested in a clinical setting.
Renal cell cancer
Fewer studies on SINEs are available in RCC, but published data demonstrating growth inhibition by SINE compounds were achieved through increasing nuclear localization of p21 and p53 to induce cell apoptosis (37,40).
Gynecologic cancer
The early pre-clinical studies in ovarian cancer cells were instrumental in furthering the understanding of CRM1 inhibitors (21). Ovarian cancer cells demonstrated sensitivity to the effects of selinexor alone and in combination with cisplatin (42). The growth inhibition effect was thought to be from FOXO1 targeting, which has been implicated in platinum-resistant disease. There are currently clinical trials in ovarian and endometrial cancers.
Leukemia
The activity of SINE compounds have been studied most extensively in pre-clinical and clinical trials involving acute leukemias (7,8). CRM1 levels are frequently elevated in leukemias and have been independently associated with a worse prognosis in patients with AML (27). CRM1 inhibitors target multiple mutations that play an important role in leukemogenesis, including NPM1, TP53, CEPBA, FLT3, and cKIT (7,8). NPM1 is a phosphoprotein that shuttles between the cytoplasm to the nucleus, and mutated NPM1 delocalizes to the cytoplasm to promote leukemogenesis via a p53-regulated pathway (53). In AML cell lines, SINEs induced apoptosis regardless of NPM1 mutational status, but the presence of a p53 mutation resulted in decreased responsiveness to inhibition by KPT-185 as compared to p53 wild type cells (27). MDM2 is a p53-specific ligase that degrades p53, and inhibition of MDM2 by Nutlin-3a increases nuclear and cytoplasmic levels of p53, leading to a p53-mediated cell apoptosis. The combination of Nutlin-3a with SINEs demonstrated synergistic activity in AML cells in vitro (27). SINE further downregulates FLT3 and c-KIT (28), and induces blast differentiation through regulation of CEBPA (29). In studies of ALL, selinexor has similarly demonstrated robust pre-clinical activity through inhibition of BCR-ABL and other TSPs (7,8,34).
Since these studies, several phase I/II clinical trials have emerged utilizing selinexor as a single-agent (54) and in combination with various chemotherapy regimens for AML, which have reported both tolerability and overall response rates ranging from 14–70% (54-57). The most commonly reported adverse effects of selinexor are gastrointestinal symptoms of nausea and vomiting, as well as fatigue, and electrolyte abnormalities (54,56-59). Recently, a second generation XPO1 inhibitor, KPT-8602, was developed and studied in pre-clinical models of AML (60) and ALL (61). The studies report decreased CNS penetration with resultant lower rates of anorexia and weight loss while maintaining similar potent anti-leukemic activity, which appears to offer an advantage over the first-generation SINEs (60,61).
In CLL, SINEs can restore normal regulation in the dysregulated pathways of CLL and can induce apoptosis in CLL cells both in vitro and in vivo (30).
Lymphoma
Similar to acute leukemias, the activity of SINE compounds in lymphomas have worked largely through nuclear localization of p53, downregulation of c-myc and NFkB, and activating pathways leading to cell apoptosis (7,8,31,38). A phase I clinical trial in patients with relapsed or refractory non-Hodgkin’s lymphoma found that selinexor was tolerable and resulted in an ORR of 31% (62). Several other early phase clinical trials in lymphoma are underway.
MM
The use of SINE compounds in MM has generated significant interest of late. CRM1 is overexpressed in MM cells, and knockdown studies showed that CRM1 is essential for MM cell viability (32). Hence, numerous pre-clinical studies followed combining SINE compounds with standard anti-MM agents that target multiple pathways, such as p53, APC, pRB, FOXO, NFkB, glucocorticoid receptor, TOP2A, and DDR (7,8,32). The consequence of CRM1 inhibition is MM cell apoptosis through activation of p53 and caspases, nuclear localization of TSPs (p53, p21, p27, Rb), downregulation of c-myc and cell cycle regulatory genes, and promotion of G1/S cell cycle arrest, just to name a few of the molecular effects (32).
There are several phase I/II clinical trials investigating selinexor in combination with other standard MM agents on patients with relapsed or refractory MM (32). Among them was one recent multicenter phase I study in heavily pre-treated patients with MM or Waldenstrom macroglobulinemia which found tolerable toxicities and an ORR of 4% when used as a single agent, and 50% when combined with dexamethasone (63). A recently published phase IIb (STORM) study of patients with refractory MM demonstrated that combining selinexor with low-dose dexamethasone achieved an ORR of 21% in quad-refractory patients and 20% in penta-refractory patients (64).
The promising future of CRM1 inhibitors
Few classes of anti-cancer agents have as broad applicability across malignancies as do the CRM1 inhibitors. This can be explained by the fact that inhibition of CRM1 targets many of the hallmark pathways of oncogenesis. Moreover, SINE compounds can be easily combined with existing standard regimens for various malignancies and have been generally well tolerated in multiple early phase clinical trials. While SINE compounds as a single agent have not demonstrated adequate potency in the clinical setting (54,63), their use in combination with existing regimens or agents have often demonstrated synergistic effects across multiple malignancies (35,50,52,55-57,63,64). Karyopharm Therapeutics is currently conducting a pivotal randomized phase III (BOSTON) study combining bortezomib, selinexor, and dexamethasone in patients with refractory MM, based on phase I/II data from the STORM study. There may even be a role in for SINE compounds in combination with immunotherapy agents or cell-based therapies, although no studies are being conducted to date. However, the gastrointestinal toxicities of selinexor have been observed as a limitation to dose escalation (54). This sets the stage for further investigation into the second-generation SINE compound KPT-8602 that may improve upon selinexor (60,61). KPT-8602 offers a 30-fold less penetration across the blood-brain barrier, while maintaining similar pharmacokinetic properties to selinexor. In mice models, this resulted in less anorexia, malaise, and weight loss as compared to selinexor, suggesting that KPT-8602 can offer a better tolerability profile while maintaining comparable efficacy (60,65). Additionally, because SINEs act through covalent binding to the exportin, mutations in the Cys528 residue can render CRM1 resistant to the SINEs, as was found in fungi (6). Hence, the development of CRM1 inhibitors that can bind in a non-covalent fashion is worth investigating as a strategy to overcome this resistance (6). The potential of SINE compounds to dramatically impact treatment outcomes is promising, but it remains to be seen how they will fare in larger clinical trial settings.
Acknowledgements
None.
Footnote
Conflicts of Interest: The authors have no conflicts of interest to declare.
References
- Gravina GL, Senapedis W, McCauley D, et al. Nucleo-cytoplasmic transport as a therapeutic target of cancer. J Hematol Oncol 2014;7:85. [Crossref] [PubMed]
- Schmidt HB, Görlich D. Transport Selectivity of Nuclear Pores, Phase Separation, and Membraneless Organelles. Trends Biochem Sci 2016;41:46-61. [Crossref] [PubMed]
- Görlich D, Mattaj IW. Nucleocytoplasmic transport. Science 1996;271:1513-8. [Crossref] [PubMed]
- Ullman KS, Powers MA, Forbes DJ. Nuclear export receptors: from importin to exportin. Cell 1997;90:967-70. [Crossref] [PubMed]
- Jamali T, Jamali Y, Mehrbod M, et al. Nuclear Pore Complex: Biochemistry and Biophysics of Nucleocytoplasmic Transport in Health and Disease. Int Rev Cell Mol Biol 2011;287:233-86. [Crossref] [PubMed]
- Sun Q, Chen X, Zhou Q, et al. Inhibiting cancer cell hallmark features through nuclear export inhibition. Signal Transduct Target Ther 2016;1:16010. [Crossref] [PubMed]
- Das A, Wei G, Parikh K, et al. Selective inhibitors of nuclear export (SINE) in hematological malignancies. Exp Hematol Oncol 2015;4:7. [Crossref] [PubMed]
- Parikh K, Cang S, Sekhri A, et al. Selective inhibitors of nuclear export (SINE)--a novel class of anti-cancer agents. J Hematol Oncol 2014;7:78. [Crossref] [PubMed]
- Dong X, Biswas A, Süel KE, et al. Structural basis for leucine-rich nuclear export signal recognition by CRM1. Nature 2009;458:1136-41. [Crossref] [PubMed]
- Fung HYJ, Chook YM. Atomic basis of CRM1-cargo recognition, release and inhibition. Semin Cancer Biol 2014;27:52-61. [Crossref] [PubMed]
- Senapedis WT, Baloglu E, Landesman Y. Clinical translation of nuclear export inhibitors in cancer. Semin Cancer Biol 2014;27:74-86. [Crossref] [PubMed]
- Stade K, Ford CS, Guthrie C, et al. Exportin 1 (Crm1p) is an essential nuclear export factor. Cell 1997;90:1041-50. [Crossref] [PubMed]
- Xu D, Grishin NV, Chook YM. NESdb: a database of NES-containing CRM1 cargoes. Mol Biol Cell 2012;23:3673-6. [Crossref] [PubMed]
- Forbes DJ, Travesa A, Nord MS, et al. Nuclear transport factors: global regulation of mitosis. Curr Opin Cell Biol 2015;35:78-90. [Crossref] [PubMed]
- Ho CY, Wong CH, Li HY. Perturbation of the chromosomal binding of RCC1, Mad2 and survivin causes spindle assembly defects and mitotic catastrophe. J Cell Biochem 2008;105:835-46. [Crossref] [PubMed]
- Turner JG, Sullivan DM. CRM1-mediated nuclear export of proteins and drug resistance in cancer. Curr Med Chem 2008;15:2648-55. [Crossref] [PubMed]
- Hamamoto T, Uozumi T, Beppu T. Leptomycins A and B, new antifungal antibiotics. III. Mode of action of leptomycin B on Schizosaccharomyces pombe. J Antibiot (Tokyo) 1985;38:1573-80. [Crossref] [PubMed]
- Kudo N, Wolff B, Sekimoto T, et al. Leptomycin B inhibition of signal-mediated nuclear export by direct binding to CRM1. Exp Cell Res 1998;242:540-7. [Crossref] [PubMed]
- Lu C, Shao C, Cobos E, et al. Chemotherapeutic sensitization of leptomycin B resistant lung cancer cells by pretreatment with doxorubicin. Abdel-Naim AB, ed. PLoS One 2012;7:e32895.
- Newlands ES, Rustin GJ, Brampton MH. Phase I trial of elactocin. Br J Cancer 1996;74:648-9. [Crossref] [PubMed]
- Liu X, Chong Y, Liu H, et al. Novel reversible selective inhibitor of CRM1 for targeted therapy in ovarian cancer. J Ovarian Res 2015;8:35. [Crossref] [PubMed]
- Sun Q, Carrasco YP, Hu Y, et al. Nuclear export inhibition through covalent conjugation and hydrolysis of Leptomycin B by CRM1. Proc Natl Acad Sci 2013;110:1303-8. [Crossref] [PubMed]
- Puente XS, Pinyol M, Quesada V, et al. Whole-genome sequencing identifies recurrent mutations in chronic lymphocytic leukaemia. Nature 2011;475:101-5. [Crossref] [PubMed]
- Jardin F, Pujals A, Pelletier L, et al. Recurrent mutations of the exportin 1 gene (XPO1) and their impact on selective inhibitor of nuclear export compounds sensitivity in primary mediastinal B-cell lymphoma. Am J Hematol 2016;91:923-30. [Crossref] [PubMed]
- García-Santisteban I, Arregi I, Alonso-Mariño M, et al. A cellular reporter to evaluate CRM1 nuclear export activity: functional analysis of the cancer-related mutant E571K. Cell Mol Life Sci 2016;73:4685-99. [Crossref] [PubMed]
- Neggers JE, Vanstreels E, Baloglu E, et al. Heterozygous mutation of cysteine in XPO1 is sufficient for resistance to selective inhibitors of nuclear export. Oncotarget 2016;7:68842-50. [Crossref] [PubMed]
- Kojima K, Kornblau SM, Ruvolo V, et al. Prognostic impact and targeting of CRM1 in acute myeloid leukemia. Blood 2013;121:4166-74. [Crossref] [PubMed]
- Ranganathan P, Yu X, Na C, et al. Preclinical activity of a novel CRM1 inhibitor in acute myeloid leukemia. Blood 2012;120:1765-73. [Crossref] [PubMed]
- Seipel K, Marques MT, Bozzini MA, et al. Inactivation of the p53-KLF4-CEBPA Axis in Acute Myeloid Leukemia. Clin Cancer Res 2016;22:746-56. [Crossref] [PubMed]
- Lapalombella R, Sun Q, Williams K, et al. Selective inhibitors of nuclear export show that CRM1/XPO1 is a target in chronic lymphocytic leukemia. Blood 2012;120:4621-34. [Crossref] [PubMed]
- Han X, Wang J, Shen Y, et al. CRM1 as a new therapeutic target for non-Hodgkin lymphoma. Leuk Res 2015;39:38-46. [Crossref] [PubMed]
- Gandhi UH, Senapedis W, Baloglu E, et al. Clinical Implications of Targeting XPO1-mediated Nuclear Export in Multiple Myeloma. Clin Lymphoma Myeloma Leuk 2018;18:335-45. [Crossref] [PubMed]
- Cheng Y, Holloway MP, Nguyen K, et al. XPO1 (CRM1) Inhibition Represses STAT3 Activation to Drive a Survivin-Dependent Oncogenic Switch in Triple-Negative Breast Cancer. Mol Cancer Ther 2014;13:675-86. [Crossref] [PubMed]
- Walker CJ, Oaks JJ, Santhanam R, et al. Preclinical and clinical efficacy of XPO1/CRM1 inhibition by the karyopherin inhibitor KPT-330 in Ph+ leukemias. Blood 2013;122:3034-44. [Crossref] [PubMed]
- Sun H, Hattori N, Chien W, et al. KPT-330 has antitumour activity against non-small cell lung cancer. Br J Cancer 2014;111:281-91. [Crossref] [PubMed]
- Yang J, Bill MA, Young GS, et al. Novel Small Molecule XPO1/CRM1 Inhibitors Induce Nuclear Accumulation of TP53, Phosphorylated MAPK and Apoptosis in Human Melanoma Cells. Haass NK, ed. PLoS One 2014;9:e102983.
- Inoue H, Kauffman M, Shacham S, et al. CRM1 blockade by selective inhibitors of nuclear export attenuates kidney cancer growth. J Urol 2013;189:2317-26. [Crossref] [PubMed]
- Yoshimura M, Ishizawa J, Ruvolo V, et al. Induction of p53-mediated transcription and apoptosis by exportin-1 (XPO1) inhibition in mantle cell lymphoma. Cancer Sci 2014;105:795-801. [Crossref] [PubMed]
- Azmi AS, Aboukameel A, Bao B, et al. Selective Inhibitors of Nuclear Export Block Pancreatic Cancer Cell Proliferation and Reduce Tumor Growth in Mice. Gastroenterology 2013;144:447-56. [Crossref] [PubMed]
- Wettersten HI, Landesman Y, Friedlander S, Shacham S, Kauffman M, Weiss RH. Specific inhibition of the nuclear exporter exportin-1 attenuates kidney cancer growth. Chandra D, ed. PLoS One 2014;9:e113867.
- Azmi AS, Al-Katib A, Aboukameel A, et al. Selective inhibitors of nuclear export for the treatment of non-Hodgkin’s lymphomas. Haematologica 2013;98:1098-106. [Crossref] [PubMed]
- Corno C, Stucchi S, De Cesare M, et al. FoxO-1 contributes to the efficacy of the combination of the XPO1 inhibitor selinexor and cisplatin in ovarian carcinoma preclinical models. Biochem Pharmacol 2018;147:93-103. [Crossref] [PubMed]
- Ranganathan P, Kashyap T, Yu X, et al. XPO1 Inhibition Using Selinexor Synergizes With Chemotherapy in Acute Myeloid Leukemia (AML) by Targeting DNA Repair and Restoring Topoisomerase II to the Nucleus. Clin Cancer Res 2016;22:6142-52. [Crossref] [PubMed]
- Search of: selinexor - List Results. c2018. ClinicalTrials.gov. Accessed June 20, 2018. Available online: https://clinicaltrials.gov/ct2/results?cond=&term=selinexor&cntry=&state=&city=&dist=
- Wang S, Han X, Wang J, et al. Antitumor effects of a novel chromosome region maintenance 1 (CRM1) inhibitor on non-small cell lung cancer cells in vitro and in mouse tumor xenografts. Xie J, ed. PLoS One 2014;9:e89848.
- Sharma SV, Bell DW, Settleman J, et al. Epidermal growth factor receptor mutations in lung cancer. Nat Rev Cancer 2007;7:169-81. [Crossref] [PubMed]
- Stewart DJ. Tumor and host factors that may limit efficacy of chemotherapy in non-small cell and small cell lung cancer. Crit Rev Oncol Hematol 2010;75:173-234. [Crossref] [PubMed]
- Gupta A, Saltarski JM, White MA, et al. Therapeutic Targeting of Nuclear Export Inhibition in Lung Cancer. J Thorac Oncol 2017;12:1446-50. [Crossref] [PubMed]
- Arango NP, Yuca E, Zhao M, et al. Selinexor (KPT-330) demonstrates anti-tumor efficacy in preclinical models of triple-negative breast cancer. Breast Cancer Res 2017;19:93. [Crossref] [PubMed]
- Kazim S, Malafa MP, Coppola D, et al. Selective Nuclear Export Inhibitor KPT-330 Enhances the Antitumor Activity of Gemcitabine in Human Pancreatic Cancer. Mol Cancer Ther 2015;14:1570-81. [Crossref] [PubMed]
- Pathria G, Wagner C, Wagner SN. Inhibition of CRM1-Mediated Nucleocytoplasmic Transport: Triggering Human Melanoma Cell Apoptosis by Perturbing Multiple Cellular Pathways. J Invest Dermatol 2012;132:2780-90. [Crossref] [PubMed]
- Salas Fragomeni RA, Chung HW, Landesman Y, et al. CRM1 and BRAF Inhibition Synergize and Induce Tumor Regression in BRAF-Mutant Melanoma. Mol Cancer Ther 2013;12:1171-9. [Crossref] [PubMed]
- Luo J, Qi C, Xu W, et al. Cytoplasmic expression of nucleophosmin accurately predicts mutation in the nucleophosmin gene in patients with acute myeloid leukemia and normal karyotype. Am J Clin Pathol 2010;133:34-40. [Crossref] [PubMed]
- Garzon R, Savona M, Baz R, et al. A phase 1 clinical trial of single-agent selinexor in acute myeloid leukemia. Blood 2017;129:3165-74. [Crossref] [PubMed]
- Alexander TB, Lacayo NJ, Choi JK, et al. Phase I study of selinexor, a selective inhibitor of nuclear export, in combination with fludarabine and cytarabine, in pediatric relapsed or refractory acute leukemia. J Clin Oncol 2016;34:4094-101. [Crossref] [PubMed]
- Fiedler W, Heuser M, Chromik J, et al. Phase II Results of Ara-C and Idarubicin in Combination with the Selective Inhibitor of Nuclear Export (SINE) Compound Selinexor (KPT-330) in Patients with Relapsed or Refractory AML. Blood 2016;128:341.
- Wang AY, Weiner H, Green M, et al. A phase I study of selinexor in combination with high-dose cytarabine and mitoxantrone for remission induction in patients with acute myeloid leukemia. J Hematol Oncol 2018;11:4. [Crossref] [PubMed]
- Abdul Razak AR, Mau-Soerensen M, Gabrail NY, et al. First-in-Class, First-in-Human Phase I Study of Selinexor, a Selective Inhibitor of Nuclear Export, in Patients With Advanced Solid Tumors. J Clin Oncol 2016;34:4142-50. [Crossref] [PubMed]
- Kuruvilla J, Byrd JC, Flynn JM, et al. The Oral Selective Inhibitor of Nuclear Export (SINE) Selinexor (KPT-330) Demonstrates Broad and Durable Clinical Activity in Relapsed/Refractory Non Hodgkin’s Lymphoma (NHL). Blood 2014;124.
- Etchin J, Berezovskaya A, Conway AS, et al. KPT-8602, a second-generation inhibitor of XPO1-mediated nuclear export, is well tolerated and highly active against AML blasts and leukemia-initiating cells. Leukemia 2017;31:143-50. [Crossref] [PubMed]
- Vercruysse T, De Bie J, Neggers JE, et al. The Second-Generation Exportin-1 Inhibitor KPT-8602 Demonstrates Potent Activity against Acute Lymphoblastic Leukemia. Clin Cancer Res 2017;23:2528-41. [Crossref] [PubMed]
- Kuruvilla J, Savona M, Baz R, et al. Selective inhibition of nuclear export with selinexor in patients with non-Hodgkin lymphoma. Blood 2017;129:3175-83. [Crossref] [PubMed]
- Chen C, Siegel D, Gutierrez M, et al. Safety and efficacy of selinexor in relapsed or refractory multiple myeloma and Waldenstrom macroglobulinemia. Blood 2018;131:855-63. [Crossref] [PubMed]
- Vogl DT, Dingli D, Cornell RF, et al. Selective Inhibition of Nuclear Export With Oral Selinexor for Treatment of Relapsed or Refractory Multiple Myeloma. J Clin Oncol 2018;36:859-66. [Crossref] [PubMed]
- Hing ZA, Fung HYJ, Ranganathan P, et al. Next-generation XPO1 inhibitor shows improved efficacy and in vivo tolerability in hematological malignancies. Leukemia 2016;30:2364-72. [Crossref] [PubMed]
Cite this article as: Wang AY, Liu H. The past, present, and future of CRM1/XPO1 inhibitors. Stem Cell Investig 2019;6:6.