Epithelial-mesenchymal plasticity—engaging stemness in an interplay of phenotypes
Introduction
Cancer is a genetic disease that arises due to somatic mutations which results in a functional imbalance between tumour repressive and oncogenic signals (1). According to the World Health Organization (WHO), cancer is the second leading cause of death globally. The major cause of cancer-related death is rarely the effect of the primary tumour itself, but rather, the devastating spread of cancer cells in metastases (2).
Epithelial-mesenchymal plasticity (EMP) plays a fundamental role in cancer metastasis. EMP is termed as the ability of cells in maintaining its plasticity and transit between epithelial-mesenchymal transition (EMT) and mesenchymal-epithelial transition (MET) states (3). These cell transitions allow them migrate from primary tumour and invade at the secondary site (4). EMP is associated with migration, invasion, colonisation, self-renewable and drug resistance (4-6). Although much research has delved into the process of carcinogenesis leading to metastasis, the crux of EMP mechanisms and its microenvironment remains poorly understood. This review briefly elucidates the mechanism of EMP, the association of cancer stem cells (CSCs) and circulation tumour cells, biomarkers and signalling pathways involved in EMP as well as drug resistance and therapeutic targeting.
EMP
The ability of tumour cells to undergo EMT and the reverse differentiation process MET is termed as EMP. EMP is the dynamic flux within the spectrum of phenotypic states that invasive carcinoma cells may reside (4). EMT is significant during embryogenesis, wound healing, fibrosis and cancer metastasis as the cells transit between epithelial and mesenchymal states in a highly plastic and dynamic manner which can help in the understanding of human disease and tumour progression (7). EMT is associated with several traits, which are decreased cell-cell adhesion; alterations of cytoskeleton, tight junctions and hemidesmosomes; loss in apical-basal polarity and gain of front-rear polarity; promote migration and invasion of cells by degrading and remodelling extracellular matrix, and ultimately, colonise at the secondary site (8,9). Moreover, EMT is associated with drug resistance, evasion of the immune system and the ability to generate CSCs (4,8). Several microenvironment conditions may induce EMT, such as the activation of EMT transcription factors which include ZEB, Snail, and Twist; matrix stiffness and hypoxia (8,10). EMT transcription factors contribute to tumour progression by inducing resistance to apoptosis and maintaining stem-like properties demonstrated in recurrent tumours (10).
However, during EMT progression, cells can exhibit a hybrid epithelial/mesenchymal (E/M) phenotype, of which the cells will co-express epithelial and mesenchymal markers (11-13) (Figure 1). It has been shown that cells exhibiting this mix of phenotypes will allow them to migrate collectively during mammary gland formation, trachea development and wound healing (12,14). In addition, collective cells would also be seen in migration as CTC clusters in the bloodstream of breast, lung and prostate cancer patients (15-18). CTC clusters that co-express epithelial and mesenchymal markers can enter and exit the bloodstream more efficiently, posing a higher metastatic risk in cancer cells (11,19). Stemness is also associated with cells that adopt the hybrid E/M state (11). As the clusters reach the distant target site, they will undergo MET which allows them to regain cell-cell adhesion and exhibit an epithelial phenotype, and thus colonise secondary site forming a secondary tumour. MET is found to be an essential driver in the later stages of metastasis.
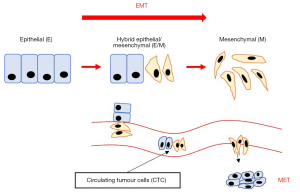
In summary, the most striking difference between these three cell states is that EMT is crucial for the transformation of a benign tumour to an invasive carcinoma; cells in the hybrid E/M state are more aggressive and responsible for the migration of clusters in bloodstream; whereas MET is crucial for the colonisation at distant sites.
CSCs and circulating tumour cells (CTCs)
EMP induces tumour cells to develop stem cell characteristics, which promote cells to invade surrounding tissues and contribute to resistance against therapeutics. These cells are inherently termed ‘cancer stem cells’. CSCs are a group of cells that are capable of self-renewal, initiate tumour generation, and undergo heterogeneous differentiation (20).
EMP also plays a critical role in disseminating tumour cells into the haemodynamic circulation, which is known as CTCs (9,21). CTCs are known as individual cells that dissolve from the primary tumour and enter the circulation during the tumour outgrowth (22). In addition, EMT facilitates the entry of CTCs into blood circulation by activation of the transforming growth factor-beta (TGF-β) pathway. CTCs are able to survive in circulation due to the presence of more mesenchymal-shifted cells in the clusters (21).
CSCs express specific cell-surface markers, namely CD24, CD44, CD133, CD200, epithelial cell adhesion molecule (EpCAM), ATP-binding cassette B5 (ABCB5) and THY1. The most significant property of CSCs is that it downregulates and upregulates the expression of CD24 and CD44 glycoproteins respectively. However, specific cancer types express unique cell surface markers and their level of expression depends on their phenotype and properties (9,23). For example, breast carcinoma cells express CD44high/CD24low, lung carcinoma has an elevated expression of CD44 and CD133, prostate carcinoma cells express EpCAMlow/CD24low, while colon carcinoma cells express CD133high/CD26low (9).
EMT biomarkers
In the course of EMT, the alteration of cellular phenotypes leads to the advent of specific biomarkers. Identification of biomarkers is clinically important in tumour diagnosis as the tumour biomarkers can be utilised in cancer patient therapy and enhance prognostic value (24). E-cadherin is a plasma membrane protein expressed by epithelial cells, which is responsible for maintaining cell adhesion as well as tight junction proteins (ZO-1).
As mentioned, when EMT is in progress, cells will lose their adhesion and polarity characteristics. Thus, there will be reduced expression of E-cadherin, and vimentin filaments will be produced (24,25). Loss of E-cadherin is said to be the hallmark of EMT (26). Mesenchymal markers such as N-cadherin, Snail, Slug, Twist, fibronectin and ZEB1 levels increase accordingly during the EMT process (27-29). An upregulation of N-cadherin expression with concomitant downregulation of E-cadherin contributes to the loss of cell-cell adhesion (30). Snail and Slug are Zinc-finger transcriptional factors which bind to the E-box of proximal E-cadherin promoter CDH1, and ultimately suppress E-cadherin transcription resulting in the disruption of cellular tight junctions (31-33).
Cytokeratin, a cytoplasmic intermediate filament protein, is the main structural element that make up the cytoskeleton in epithelial cells. It functions to maintain structural rigidity and multipurpose scaffolds. Recent studies show that cytokeratin 19 (KRT19) and CK19 is upregulated in breast cancer and lung cancer respectively (34,35). Not only that, overexpressed CK19 can be seen in most of the epithelial malignancies, such as cervical, colorectal and thyroid carcinomas. Keratin content increases when epithelial cells undergo EMT and transform into cancer cells (35).
Interestingly, extracellular vesicles also play a critical role in transitioning to mesenchymal-like cells. They help in migration between disseminated tumour cells and other cells in the tumour microenvironment by cell-cell interaction. Extracellular vesicle contents change with phenotypic alterations. Recently, many studies have shown that extracellular vesicles are associated with metastasis (24). For example, in an N-SMase2-knockout transplanted breast cancer model, the reduction in extracellular vesicles attenuated tumour metastasis (36). The reasons that highlight extracellular vesicles as ideal biomarkers for cancer prognosis and surveillance are their non-invasive sampling, high stability and sensitivity, ability to represent parent cells, and tumour-specific RNA molecules are transported in biological fluids throughout the body (24).
Regulation of EMP by microRNAs
miRNAs are small, non-encoding RNAs that regulate gene expression by binding to mRNA, leading to translational silencing and subsequently repress protein production (37,38). Several studies have shown that miRNA expression in serum extracellular vesicles may be useful as biomarkers for various cancers such as non-small-cell lung carcinoma (NSCLS), liver metastasis, chronic lymphocytic leukaemia, colorectal cancer, pancreatic cancer and tongue squamous cell carcinoma (SCC) (39-43). For instance, a low expression of miR-146a-5p is associated with higher recurrence rates in NSCLS patients compared to subjects expressing high levels (39). Other markers include miR-21, which is associated with oesophageal cancer recurrence and distant metastasis, miR-638 is correlated with liver metastasis and colorectal cancer, and miR-125b is implicated in advanced melanoma (24).
Many studies have illustrated that the modulation of miRNA expression not only contributes to tumorigenesis, metastasis, and multi-drug resistance, but also tumour response to treatment in various cancers (44-46). miRNAs target a multitude of signalling pathways and transcription factors, causing the aberrant mechanisms such as dysregulation of protein translation. A recent study showed that overexpression of miR-21 promotes invasion and migration, and decreases apoptotic effects induced by cisplatin in lung adenocarcinoma and gastric cancer cells respectively (40,47). Overexpression of miR-223 and miR-221 are shown to correlate with resistance towards Gemcitabine and 5-fluorouracil in pancreatic and oesophageal cancers respectively (48,49). Conversely, the inhibition of miR-223 reversed the EMT phenotype (49) while knockdown of miR-221 seemed to result in increased apoptosis, restored chemosensitivity and inactivation of the Wnt pathway (48).
Signalling pathways involved in EMT stimulation
EMT is induced through the activation of several signalling pathways; the three most common being TGF-β signalling, Wnt-β-catenin pathway and Notch. Most of the time, these pathways and transcription factors will eventually lead to E-cadherin regulation by binding to the E-box of its DNA sequences (50,51).
The Wnt pathway is activated when Wnt ligands bind to seven-transmembrane receptor Frizzled (FZD) and low-density lipoprotein receptor-related protein (LRP), which subsequently accumulate and stabilise β-catenin, and then translocate into the nucleus and regulate gene expression (52,53). β-catenin is a co-activator of the TCF/LEF family that regulates Wnt target gene expression levels. Beta-catenin pathway-related genes that regulate its stability may be mutated and stimulate incessant β-catenin activity, for example in adenomatous polyposis coli (APC). Glycogen synthase kinase 3β (GSK3β) and casein kinase Iα (CKIα) upregulate levels of β-catenin in the nucleus, subsequently activating Wnt target genes (54,55). Abnormal activation of Wnt signalling is shown to induce EMT (56,57).
It is well-established that TGF-β signalling is one of the major pathways that promote EMT through the activation of SMAD family (Smad 2 and Smad 3) proteins which will translocate to nucleus and act as a cofactor in transcription of target genes (58). Several mesenchymal markers such as Snail, Slug, Twist and ZEB1 are induced through Smad signalling, which will subsequently repress E-cadherin (59). Interestingly, the Wnt pathway can cross talk with TGF-β/SMAD due to the removal of β-catenin from adherent junctions in a process that involves TGF-β-dependent PTEN dissociation from β-catenin and Akt activation (60).
The Notch signalling pathway is activated when Notch, a transmembrane receptor binds to the transmembrane ligands, Delta and Jagged. Ligand binding causes cleavage in Notch to release Notch intra-cellular domain (NICD) which will then enter the nucleus and activate the Notch pathway (61-63). Studies have shown that Notch activity downregulates E-cadherin and upregulates Snail (64,65). A recent study has revealed an inhibitor of the Notch signalling pathway—Numb, which can essentially prevent cells from undergoing a full transition into the mesenchymal phenotype (66).
Further investigations are indispensable to unravel the complex signalling pathways that drive EMT. Blocking or inactivating specific pathways may prove useful to restrain EMT from occurring and eventually prevent tumorigenesis, invasion and migration of cancer cells.
Drug resistance and therapeutic targeting in EMT
EMT is well-studied in that the process itself, in the presence of CSCs, not only show resistance to apoptotic stimuli, but also contribute to drug resistance (35,67-69). EMT-induced CSCs that are mediated by the FGFR signalling pathway activation are more resistant to drugs (70). Gene mutations, abnormal cell cycle regulation and DNA repair, and long-term drug administration induce multi-drug resistance (MDR) and cause tumour cells to exhibit EMT-associated phenotypic changes in cellular morphology and also enrich the CD44+/CD24− stem cell population (71-73). A breast cancer cell line, MDA-MB-231, and advanced hepatocellular carcinoma cells (HCCs) were shown to have resistance towards epirubicin and sorafenib respectively after long term exposure (71,74).
Evidence show that over-expression of GNA13 contributes to drug resistance in head and neck squamous cell carcinoma (HNSCC). To overcome this, inhibition of GNA13-induced signalling effectively alleviated drug resistance (75). Luo et al. (in 2018) reported that upregulation of 14,15-epoxyeicosatrienoic acid (14,15-EET) induces EMT in breast cancer cells and confer cisplatin resistance. In contrast, inhibition of 14,15-EET may restore cisplatin sensitivity (76). Lee et al. (in 2018) discovered that combining HNHA (histone deacetylase) and lenvatinib in treating patient-derived thyroid cancer cells has a significant effect by blocking the FGFR signalling pathway, and thus inhibit EMT (70). Some examples of tumour-specific targeted therapeutic regimens are itemised in Table 1.
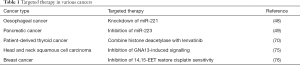
Full table
Conclusions
In summary, EMP has cemented its pivotal role in cancer progression. This review summarised the association between CSCs and EMT, signalling pathways that drive EMT, potential biomarkers of EMT, drug resistance and possible targeting therapeutic treatment in various cancers. Much effort has been made by researchers to obtain a more in-depth understanding of the mechanism of EMT and MET, as well as their association with CSCs. Future work could be focused on elucidating different biomarkers for early prognosis of cancer; silencing signalling pathways that drive EMT and possibly prevent cancer metastasis; and evoke further understanding of miRNAs as potential targeted therapy to overcome chemo-resistance in various cancers.
Acknowledgments
The authors would like to thank the authorities of International Medical University, Malaysia, for the provision of necessary facilities in the preparation of this manuscript.
Funding: This work was supported by the Fundamental Research Grant Scheme (FRGS/1/2016/SKK08/IMU/03/2) from the Ministry of Education, Malaysia.
Footnote
Conflicts of Interest: The authors have no conflicts of interest to declare.
Ethical Statement: The authors are accountable for all aspects of the work in ensuring that questions related to the accuracy or integrity of any part of the work are appropriately investigated and resolved.
References
- Vogelstein B, Papadopoulos N, Velculescu VE, et al. Cancer genome landscapes. Science 2013;339:1546-58. [Crossref] [PubMed]
- Plummer M, de Martel C, Vignat J, et al. Global burden of cancers attributable to infections in 2012: a synthetic analysis. Lancet Glob Health 2016;4:e609-16. [Crossref] [PubMed]
- Luo M, Brooke M, Wicha MS. Epithelial-Mesenchymal Plasticity of Breast Cancer Stem Cells: Implications for Metastasis and Therapeutic Resistance. Curr Pharm Des 2015;21:1301-10. [Crossref] [PubMed]
- Bhatia S, Monkman J, Toh AKL, et al. Targeting epithelial-mesenchymal plasticity in cancer: clinical and preclinical advances in therapy and monitoring. Biochem J 2017;474:3269-306. [Crossref] [PubMed]
- Diepenbruck M, Christofori G. Epithelial-mesenchymal transition (EMT) and metastasis: Yes, no, maybe? Curr Opin Cell Biol 2016;43:7-13. [Crossref] [PubMed]
- Tsai JH, Yang J. Epithelial - mesenchymal plasticity in carcinoma metastasis. Genes Dev 2013;27:2192-206. [Crossref] [PubMed]
- Nieto MA, Huang RYJ, Jackson RA, et al. EMT: 2016. Cell 2016;166:21-45. [Crossref] [PubMed]
- Jolly MK, Preca BT, Tripathi SC, et al. Interconnected feedback loops among ESRP1, HAS2, and CD44 regulate epithelial-mesenchymal plasticity in cancer. APL Bioeng 2018;2:031908. [Crossref] [PubMed]
- Shibue T, Weinberg RA. EMT, CSCs, and drug resistance: The mechanistic link and clinical implications. Nat Rev Clin Oncol 2017;14:611-29. [Crossref] [PubMed]
- Runyan RB, Savagner P. Epithelial-mesenchymal transition and plasticity in the developmental basis of cancer and fibrosis. Dev Dyn 2018;247:330-1. [Crossref] [PubMed]
- Grosse-Wilde A, Fouquier D’hérouël A, et al. Stemness of the hybrid Epithelial/ Mesenchymal State in Breast Cancer and Its Association with Poor Survival. PLoS One 2015 8;10:e0126522.
- Micalizzi DS, Farabaugh SM, Ford HL, et al. Epithelial-Mesenchymal Transition in Cancer: Parallels Between Normal Development and Tumor Progression-β Transforming Growth Factor-β FGF Fibroblast Growth Factor BMP Bone Morphogenetic Protein MEE Medial Edge Epithelium TEB Terminal End Bud EGF Epiderma. J Mammary Gland Biol Neoplasia 2010;15:117-34. [Crossref] [PubMed]
- Yu M, Bardia A, Wittner BS, Stott SL, et al. Circulating breast tumor cells exhibit dynamic changes in epithelial and mesenchymal composition. Science 2013;339:580-4. [Crossref] [PubMed]
- Revenu C, Gilmour D. EMT 2.0: shaping epithelia through collective migration. Curr Opin Genet Dev 2009;19:338-42. [Crossref] [PubMed]
- Christiansen JJ, Rajasekaran AK. Reassessing epithelial to mesenchymal transition as a prerequisite for carcinoma invasion and metastasis. Cancer Res 2006;66:8319-26. [Crossref] [PubMed]
- Lecharpentier A, Vielh P, Perez-Moreno P, et al. Detection of circulating tumour cells with a hybrid (epithelial/mesenchymal) phenotype in patients with metastatic non-small cell lung cancer. Br J Cancer 2011;105:1338-41. [Crossref] [PubMed]
- Hou JM, Krebs M, Ward T, et al. Circulating tumor cells as a window on metastasis biology in lung cancer. Am J Pathol 2011;178:989-96. [Crossref] [PubMed]
- Armstrong AJ, Marengo MS, Oltean S, et al. Circulating tumor cells from patients with advanced prostate and breast cancer display both epithelial and mesenchymal markers. Mol Cancer Res 2011;9:997-1007. [Crossref] [PubMed]
- Joosse SA, Gorges TM, Pantel K. Biology, detection, and clinical implications of circulating tumor cells. EMBO Mol Med 2015;7:1-11. [Crossref] [PubMed]
- Cai Z, Cao Y, Luo Y, et al. Signalling mechanism(s) of epithelial-mesenchymal transition and cancer stem cells in tumour therapeutic resistance. Clin Chim Acta 2018;483:156-63. [Crossref] [PubMed]
- Francart ME, Lambert J, Vanwynsberghe AM, et al. Epithelial-mesenchymal plasticity and circulating tumor cells: Travel companions to metastases. Dev Dyn 2018;247:432-50. [Crossref] [PubMed]
- Kölbl AC, Jeschke U, Andergassen U. The Significance of Epithelial-to-Mesenchymal Transition for Circulating Tumor Cells. Int J Mol Sci 2016;17:1308. [Crossref] [PubMed]
- Phi LTH, Sari IN, Yang YG, et al. Cancer Stem Cells (CSCs) in Drug Resistance and their Therapeutic Implications in Cancer Treatment. Stem Cells Int 2018;2018:5416923. [Crossref] [PubMed]
- Sun YZ, Ruan JS, Jiang ZS, et al. Extracellular Vesicles: A New Perspective in Tumor Therapy. Biomed Res Int 2018;2018:2687954. [Crossref] [PubMed]
- Morris HT, Machesky LM. Actin cytoskeletal control during epithelial to mesenchymal transition: focus on the pancreas and intestinal tract. Br J Cancer 2015;112:613-20. [Crossref] [PubMed]
- Adhikary A, Chakraborty S, Mazumdar M, et al. Inhibition of epithelial to mesenchymal transition by E-cadherin up-regulation via repression of slug transcription and inhibition of E-cadherin degradation: dual role of scaffold/matrix attachment region-binding protein 1 (SMAR1) in breast cancer cells. J Biol Chem 2014;289:25431-44. [Crossref] [PubMed]
- Gonzalez DM, Medici D. Signaling mechanisms of the epithelial-mesenchymal transition. Sci Signal 2014;7:re8. [Crossref] [PubMed]
- Suyama K, Shapiro I, Guttman M, et al. A signaling pathway leading to metastasis is controlled by N-cadherin and the FGF receptor. Cancer Cell 2002;2:301-14. [Crossref] [PubMed]
- Ganesan R, Mallets E, Gomez-Cambronero J. The transcription factors Slug (SNAI2) and Snail (SNAI1) regulate phospholipase D (PLD) promoter in opposite ways towards cancer cell invasion. Mol Oncol 2016;10:663-76. [Crossref] [PubMed]
- Onder TT, Gupta PB, Mani SA, et al. Loss of E-cadherin promotes metastasis via multiple downstream transcriptional pathways. Cancer Res 2008;68:3645-54. [Crossref] [PubMed]
- Seki K, Fujimori T, Savagner P, et al. Mouse Snail family transcription repressors regulate chondrocyte, extracellular matrix, type II collagen, and aggrecan. J Biol Chem 2003;278:41862. [Crossref] [PubMed]
- Molina-Ortiz P, Villarejo A, MacPherson M, et al. Characterization of the SNAG and SLUG Domains of Snail2 in the Repression of E-Cadherin and EMT Induction: Modulation by Serine 4 Phosphorylation. PLoS One 2012;7:e36132. [Crossref] [PubMed]
- Baranwal S, Alahari SK. Molecular mechanisms controlling E-cadherin expression in breast cancer. Biochem Biophys Res Commun 2009;384:6-11. [Crossref] [PubMed]
- Saha SK, Kim K, Yang GM, et al. Cytokeratin 19 (KRT19) has a Role in the Reprogramming of Cancer Stem Cell-Like Cells to Less Aggressive and More Drug-Sensitive Cells. Int J Mol Sci 2018. [Crossref] [PubMed]
- Barillo JL, da Silva CT Junior, Silva PS, et al. Increased Cytokeratin 19 Fragment Levels Are Positively Correlated with Adenosine Deaminase Activity in Malignant Pleural Effusions from Adenocarcinomas. Dis Markers 2018;2018:2609767. [Crossref] [PubMed]
- Kosaka N, Iguchi H, Hagiwara K, et al. Neutral sphingomyelinase 2 (nSMase2)-dependent exosomal transfer of angiogenic microRNAs regulate cancer cell metastasis. J Biol Chem 2013;288:10849-59. [Crossref] [PubMed]
- Peng Y, Croce CM. The role of MicroRNAs in human cancer. Signal Transduct Target Ther 2016;1:15004. [Crossref] [PubMed]
- Cannell IG, Kong YW, Bushell M. How do microRNAs regulate gene expression? Biochem Soc Trans 2008;36:1224-31. [Crossref] [PubMed]
- Yuwen DL, Sheng BB, Liu J, et al. MiR-146a-5p level in serum exosomes predicts therapeutic effect of cisplatin in non-small cell lung cancer. Eur Rev Med Pharmacol Sci 2017;21:2650-8. [PubMed]
- Su C, Cheng X, Li Y, et al. MiR-21 improves invasion and migration of drug-resistant lung adenocarcinoma cancer cell and transformation of EMT through targeting HBP1. Cancer Med 2018;7:2485-503. [Crossref] [PubMed]
- Hwang JH, Voortman J, Giovannetti E, et al. Identification of microRNA-21 as a biomarker for chemoresistance and clinical outcome following adjuvant therapy in resectable pancreatic cancer. PLoS One 2010;5:e10630. [Crossref] [PubMed]
- Rossi S, Shimizu M, Barbarotto E, et al. microRNA fingerprinting of CLL patients with chromosome 17p deletion identify a miR-21 score that stratifies early survival. Blood 2010;116:945-52. [Crossref] [PubMed]
- Xie Y, Todd NW, Liu Z, et al. Altered miRNA expression in sputum for diagnosis of non-small cell lung cancer. Lung Cancer 2010;67:170-6. [Crossref] [PubMed]
- Hahne JC, Valeri N. Non-Coding RNAs and Resistance to Anticancer Drugs in Gastrointestinal Tumors. Front Oncol 2018;8:226. [Crossref] [PubMed]
- Hu W, Tan C, He Y, et al. Functional miRNAs in breast cancer drug resistance. Onco Targets Ther 2018;11:1529-41. [Crossref] [PubMed]
- Xu L, Beckebaum S, Iacob S, et al. MicroRNA-101 inhibits human hepatocellular carcinoma progression through EZH2 downregulation and increased cytostatic drug sensitivity. J Hepatol 2014;60:590-8. [Crossref] [PubMed]
- Yang SM, Huang C, Li XF, et al. miR-21 confers cisplatin resistance in gastric cancer cells by regulating PTEN. Toxicology 2013;306:162-8. [Crossref] [PubMed]
- Wang Y, Zhao Y, Herbst A, et al. MiR-221 mediates chemoresistance of esophageal adenocarcinoma by direct targeting of DKK2 expression. Ann Surg 2016;264:804-14. [Crossref] [PubMed]
- Ma J, Fang B, Zeng F, et al. Down-regulation of miR-223 reverses epithelial-mesenchymal transition in gemcitabine-resistant pancreatic cancer cells. Oncotarget 2015;6:1740-9. [Crossref] [PubMed]
- Hofman P, Vouret-Craviari V. Microbes-induced EMT at the crossroad of inflammation and cancer. Gut Microbes 2012;3:176-85. [Crossref] [PubMed]
- Lamouille S, Xu J, Derynck R. Molecular mechanisms of epithelial-mesenchymal transition. Nat Rev Mol Cell Biol 2014;15:178. [Crossref] [PubMed]
- Nusse R, Clevers H. Wnt/β-Catenin Signaling, Disease, and Emerging Therapeutic Modalities. Cell 2017;169:985-99. [Crossref] [PubMed]
- Mitra T, Roy SS. Co-Activation of TGFβ and Wnt Signalling Pathways Abrogates EMT in Ovarian Cancer Cells. Cell Physiol Biochem 2017;41:1336-45. [Crossref] [PubMed]
- Clevers H. Wnt/β-Catenin Signaling in Development and Disease. Cell 2006;127:469-80. [Crossref] [PubMed]
- MacDonald BT, Tamai K, He X. Wnt/beta-catenin signaling: components, mechanisms, and diseases. Dev Cell 2009;17:9-26. [Crossref] [PubMed]
- Gasior K, Hauck M, Wilson A, et al. A Theoretical Model of the Wnt Signaling Pathway in the Epithelial Mesenchymal Transition. Theor Biol Med Model 2017;14:19. [Crossref] [PubMed]
- Anastas JN, Moon RT. WNT signalling pathways as therapeutic targets in cancer. Nat Rev Cancer 2013;13:11. [Crossref] [PubMed]
- Lebrun JJ. The Dual Role of TGF β in Human Cancer: From Tumor Suppression to Cancer Metastasis. ISRN Mol Biol 2012;2012:381428. [PubMed]
- Katsuno Y, Lamouille S, Derynck R. TGF-β signaling and epithelial-mesenchymal transition in cancer progression. Curr Opin Oncol 2013;25:76-84. [Crossref] [PubMed]
- Yu M, Trobridge P, Wang Y, et al. Inactivation of TGF-β signaling and loss of PTEN cooperate to induce colon cancer in vivo. Oncogene 2014;33:1538. [Crossref] [PubMed]
- Capaccione KM, Pine SR. The Notch signaling pathway as a mediator of tumor survival. Carcinogenesis 2013;34:1420-30. [Crossref] [PubMed]
- Andersson ER, Lendahl U. Therapeutic modulation of Notch signalling — are we there yet? Nat Rev Drug Discov 2014;13:357. [Crossref] [PubMed]
- Andersson ER, Sandberg R, Lendahl U. Notch signaling: simplicity in design, versatility in function. Development 2011;138:3593-612. [Crossref] [PubMed]
- Timmerman LA, Grego-Bessa J, Raya A, et al. Notch promotes epithelial-mesenchymal transition during cardiac development and oncogenic transformation. Genes Dev 2004;18:99-115. [Crossref] [PubMed]
- Chang ACY, Garside VC, Fournier M, et al. A Notch-dependent transcriptional hierarchy promotes mesenchymal transdifferentiation in the cardiac cushion. Dev Dyn 2014;243:894-905. [Crossref] [PubMed]
- Bocci F, Jolly MK, Tripathi SC, et al. Numb prevents a complete epithelial-mesenchymal transition by modulating Notch signalling. J R Soc Interface 2017;14. [Crossref] [PubMed]
- Mittal V. Epithelial Mesenchymal Transition in Aggressive Lung Cancers. Adv Exp Med Biol 2016;890:37-56. [Crossref] [PubMed]
- Mitra A, Mishra L, Li S. EMT, CTCs and CSCs in tumor relapse and drug-resistance. Oncotarget 2015;6:10697-711. [Crossref] [PubMed]
- Prieto-Vila M, Takahashi RU, Usuba W, et al. Drug Resistance Driven by Cancer Stem Cells and Their Niche. Int J Mol Sci 2017. [Crossref] [PubMed]
- Lee YS, Kim SM, Kim BW, et al. Anti-cancer Effects of HNHA and Lenvatinib by the Suppression of EMT-Mediated Drug Resistance in Cancer Stem Cells. Neoplasia 2018;20:197-206. [Crossref] [PubMed]
- Xu X, Zhang L, He X, et al. TGF-β plays a vital role in triple-negative breast cancer (TNBC) drug-resistance through regulating stemness, EMT and apoptosis. Biochem Biophys Res Commun 2018;502:160-5. [Crossref] [PubMed]
- Housman G, Byler S, Heerboth S, et al. Drug resistance in cancer: an overview. Cancers (Basel) 2014;6:1769-92. [Crossref] [PubMed]
- Zahreddine H, Borden KLB. Mechanisms and insights into drug resistance in cancer. Front Pharmacol 2013;4:28. [Crossref] [PubMed]
- Dong J, Zhai B, Sun W, et al. Activation of phosphatidylinositol 3-kinase/AKT/snail signaling pathway contributes to epithelial-mesenchymal transition-induced multi-drug resistance to sorafenib in hepatocellular carcinoma cells. PLoS One 2017;12:e0185088. [Crossref] [PubMed]
- Rasheed SAK, Leong HS, Lakshmanan M, et al. GNA13 expression promotes drug resistance and tumor-initiating phenotypes in squamous cell cancers. Oncogene 2018;37:1340-53. [Crossref] [PubMed]
- Luo J, Yao JF, Deng XF, et al. 14, 15-EET induces breast cancer cell EMT and cisplatin resistance by up-regulating integrin αvβ3 and activating FAK/PI3K/AKT signaling. J Exp Clin Cancer Res 2018;37:23. [Crossref] [PubMed]
Cite this article as: Chin VL, Lim CL. Epithelial-mesenchymal plasticity—engaging stemness in an interplay of phenotypes. Stem Cell Investig 2019;6:25.