Protein kinase C delta (PKCδ) splice variant modulates senescence via hTERT in adipose-derived stem cells
Introduction
Mature human adipocytes possess a large, single lipid droplet and are considered to be in the terminal stage of differentiation. They have lost their proliferative ability and are stationary. Adipose stem cells that reside within the stromal vascular fraction of adipose tissue, however, encode transcription factors that suppress differentiation and allow stem cells to continue to proliferate while remaining poised to develop into cells of multiple lineages. Mesenchymal marker proteins are used to identify and characterize multipotent stem cells that can undergo this differentiation. We have isolated adipose-derived stem cells (ADSC) and characterized them using stem cell markers (1). Further, we show that in vitro differentiation of ADSC derived from lean and obese have distinct gene profiles (2).
Normal human cells have a limited replicative capacity and enter senescence. Normal cells lose 50-100 bp from their telomere ends in the chromosomes and this may lead to senescence. Telomerase maintains chromosome length by synthesizing and adding six nucleotide repeats at the end of telomeres (3). Immortalized cells and cancer cells have longer telomeres. Cells undergoing senescence have shorter telomeres. Increased telomerase activity is associated with unlimited growth potential and is routinely associated with cancers. Telomerase, a ribonucleoprotein complex, is made up of two essential components: the human telomerase RNA (hTR) which serves as a transcription template and human telomerase reverse transcriptase (hTERT) which is the catalytic subunit of telomerase and its expression controls enzymatic activity. hTERT (4) has at least 15 splice variants; however α+/β+ variant codes for the full length hTERT. hTERT splicing is tissue-specific and also depends on age (5).
Obesity changes the genetic profile of its stem cell niche. The adipocytes in obese patients reflect hyperplasia and hypertrophy. We recently published that differentiating pre-adipocytes from obese ADSC are resistant to apoptosis (2). Protein kinase C delta (PKCδ) is a serine/threonine kinase which plays a central role in apoptosis. It’s alternatively spliced variants PKCδI and PKCδVIII play opposing roles in apoptosis: PKCδI promotes apoptosis while PKCδVIII promotes survival (6). We showed that PKCδVIII expression is increased in obese adipocytes. Here, we determine the relationship between a survival kinase PKCδVIII and hTERT expression in adipose derived stem cells from a lean and obese subject.
Methods
Cell culture: human ADSC
The lean and obese ADSC were purchased as cryopreserved cells from ZenBio™ (Research Triangle Park, NC, USA). Since these commercial cell lines and their information are publicly available, they are exempt from human subjects research determination from internal review board (IRB) in accordance with HHS regulation at 45 CFR Part 46. The lean ADSC originated from subcutaneous adipose tissue of a healthy donor 26 years of age undergoing elective surgery with a body mass index 21.3. An additional obese ADSC originated from subcutaneous adipose tissue of a de-identified donor undergoing elective surgery with a body mass index 48. The sample was obtained under IRB approved exemption (#108360, University of South Florida) and the adipose stromal vascular fraction was isolated as described in our previous publication (1). The ADSC lines were tested in culture to differentiate into mature adipocytes and show accumulation of lipid and secrete adiponectin and leptin. At the start of all experiments, cells are grown to confluency such that all cells are synchronized and then differentiated. The cells were cultured as follows. Briefly, cryopreserved preadipocytes were passaged with preadipocyte medium (PM-1; DMEM/Ham’s F-12 medium, HEPES, FBS, penicillin, streptomycin, amphotericin B; Zen-Bio) and then plated 40,625 cells/cm2 with PM-1. Cells were fed every other day with PM-1 until confluent. To induce differentiation, PM-1 medium was replaced with differentiation medium (DM2; Zen-Bio) including biotin, pantothenate, human insulin, dexamethasone, isobutylmethylxanthine and a PPARγ agonist (days 0-7). After 7 days, DM-2 medium was removed and cells were incubated for an additional 7 days with Adipocyte Medium (AM1; Zen-Bio; days 7-14), which included PM-1, biotin, pantothenate, human insulin and dexamethasone. By day 14, cells contained large lipid droplets and were considered mature adipocytes. Cells were maintained at 37 ℃ in a humidified 5% CO2 atmosphere.
Transient transfection of plasmid DNA
ADSCs were trypsinized and cell pellets were collected in 100 µL Nucleofector® solution (Lonza) and combined with plasmid DNA (2 µg). The cell/DNA solution is transferred to a cuvette and the program started (0.34 kV, 960 microfarads), 500 µL of medium is added immediately and cells are gently transferred to 60 mm plates and allowed to differentiate.
RT-PCR
Total RNA was isolated from cells using RNA-BeeTM (Tel Test, Inc) as per manufacturer’s instructions, 2 µg of RNA was used to synthesize first strand cDNA using an Oligo(dT) primer and Omniscript™ kit (Qiagen). PCR was performed using 2 µL of RT reaction and Jump Start PCR Ready Mix (Sigma). The primers are listed: Human PKCδ sense primer 5'-CACTATATTCCAGAAAGAACGC-3' and PKCδVIII-specific antisense primer 5'-CCCTCCCAGATCTTGCC-3'; hTERT sense primer 5'-GCCTGAGCTGTACTTTGTCAA and antisense primer 5'-CGCAAACAGCTTGTTCTCCATGTC-3'. HPRT sense primer 5'-GAAGAGCTATTGTAATGACC-3' and antisense primer 5'-GCGACCTTGACCATCTTTG-3'. Using PKCδVIII-specific primers, PKCδVIII is 424 bp; hTERT α+/β+ is 457 bp; HPRT is 391 bp. Five-percent of products were resolved on 6% PAGE gels and detected by silver staining. The PCR reaction was optimized for linear range amplification to allow for quantification of products. Un-Scan IT™ Analysis Software (Silk Scientific) was used for densitometric analyses of bands.
Western blot analysis
Protein lysates were obtained from the cells using lysis buffer containing protease inhibitors. The lysates (40 µg) were separated by SDS-PAGE with 10% gels, electrophoretically transferred to nitrocellulose membranes, blocked with Tris-buffered saline containing 0.1% Tween 20 and 5% nonfat dried milk, washed, and incubated with antibody specific for PKCδVIII [Patel lab (6)], anti-telomerase (Abcam) and β-actin (Sigma). After incubation with anti-rabbit IgG-HRP, enhanced chemiluminescence (Pierce) was used for detection. The blots were analyzed on ProteinSimple Fluor M and Alpha view™ software was used for densitometric analysis.
Gel based TRAPeze telomerase detection
We used TRAPeze kit from Chemicon (catalog number S7700). Briefly, the cells were suspended in 1× CHAPS lysis buffer. In separate tubes, the positive control was suspended in 1× CHAPS buffer. Following telomerase extension at 30 ℃ for 30 minutes, PCR is performed using the TS primer (substrate oligonucleotide) and TRAP primer mix provided in the kit. Following 30 cycles of PCR amplification, the samples were separated on PAGE gel and bands detected by silver staining.
Senescence
ADSC were grown in 60 mm plates. Cells were washed with 1× PBS and fixed with 1× fixative solution (20% formaldehyde, 2% glutaraldehyde in 10× PBS) for 15 minutes at room temperature. Cells were rinsed and 1 mL of β-galactosidase staining solution (400 mM citric acid/sodium phosphate, 1.5 M NaCl, 20 mM MgCl2, 500 mM potassium ferrocyanide and 20 mg/mL X-gal in DMF) was added to cells and incubated overnight in a dry incubator. The images were captured brightfield on a Nikon Eclipse fluorescent microscope using 4× or 10× objective with 0.38/µM pixel.
Statistical analysis
Analyses were performed using PRISM™ software and analyzed using two-tailed Student’s t-test. P<0.05 was significant; P<0.001 was highly significant; P<0.001 was extremely significant. Analysis was performed within and between groups.
Results
Telomerase expression in lean and obese differentiating preadipocytes
We isolated subcutaneous ADSC from a morbidly obese patient (1), characterized its stem cell markers and differentiated them to adipocytes in vitro. We obtained subcutaneous ADSC from a lean subject (ZenBio) and simultaneously differentiated them into adipocytes. Differentiation was initiated when the preadipocytes were 100% confluent. By day 7, mature adipocytes were formed and were maintained in adipocyte media. Since our previous results indicated that obese adipocytes were resistant to apoptosis (2), we measured the telomerase protein in differentiating lean and obese preadipocytes. We observed an increase in telomerase in differentiating obese ADSC using western blot analysis compared to lean on days 0, 4, 8 (Figure 1). Obese samples showed increased levels of PKCδVIII on day 0. This increase was concurrent to the increase in PKCδVIII expression reported previously by our lab (2).
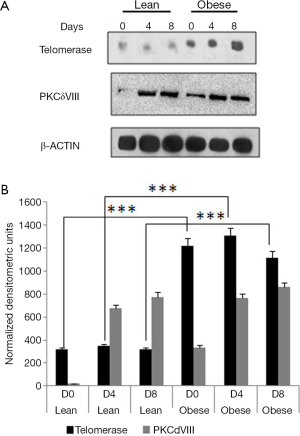
Telomeric repeat amplification protocol (TRAP) assay
We performed the TRAPeze assay (kit from Chemicon) to determine telomerase activity in these obese and lean preadipocytes. Telomerase adds a number of telomeric repeats (GGTTAG) to the 3' end of the substrate oligonucleotide TS. We assessed processivity of telomerase by measuring telomere length. The special design of the anchored reverse primer (RP) allows assessment of the processivity of telomerase in the sample extracts. The largest band of the ladder observed in the gel corresponds to the largest TS-extended product generated during the initial telomerase reaction. For a telomerase-extended product to be amplified by TS and RP primers, it must have at least three telomeric repeats. Figure 2 shows six bands in obese ADSC indicating that at least nine telomeric repeats were added while lean ADSC had one band indicating that at least four telomeric repeats were added; positive control has ten bands indicating that at least 13 telomeric repeats were added. Our data indicated an increase in addition of telomere repeats in the obese preadipocytes compared to lean.
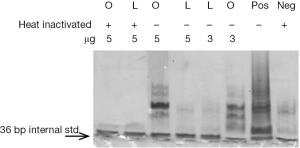
hTERT splice variants in obesity
hTERT is a rate limiting step in telomerase activation. Several alternatively spliced variants of hTERT are reported: full length α+/β+ is usually associated with active telomerase. We compared the expression of hTERT splice variants from differentiating lean and obese preadipocytes using RT-PCR with hTERT primers that simultaneously detect α+/β+, α+/β–, α–/β+ and α–/β–. Our data (Figure 3A) indicated that obese adipocytes had increased expression of α+/β+ splice variant while its expression was extremely low in lean adipocytes. Expression of other splice variants was barely detected in either lean or obese samples.
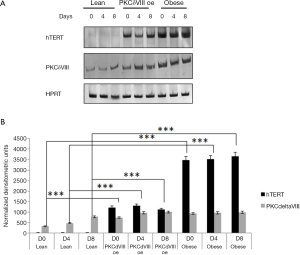
Effect of PKCδVIII on hTERT expression
We sought to determine whether PKCδVIII expression could affect hTERT splice variant expression since increased PKCδVIII expression was associated with the obese phenotype. Further, PKCδVIII is a survival protein and inhibits apoptosis. We transfected 2 µg of PKCδVIII in lean ADSC and differentiated the preadipocytes to mature adipocytes. We determined the levels of hTERT splice variants on days 0, 4 and 8. Our results (Figure 3A,B) show an increase in hTERT α+/β+ splice variant.
Effect of PKCδVIII on telomerase expression
We next determined whether PKCδVIII over-expression affected the levels of telomerase. In separate experiments, we transfected 2 µg of PKCδVIII in lean ADSC and differentiated the preadipocytes to mature adipocytes. Whole cell lysates were analyzed and immunoblotted for telomerase. Our results (Figure 4) indicate an increase in telomerase with PKCδVIII over-expression.
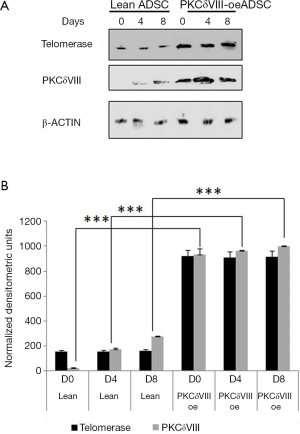
Senescence
Diploid cells lose their ability to differentiate and normal cellular senescence sets in. This is often times related to shortening of the telomeric length. PKCδ has been shown to affect cellular senescence (7), however the splice variant specificity is not elucidated. Since, our data indicated that over-expression of PKCδVIII increased telomerase, we evaluated its effect on senescence. We simultaneously evaluated senescence in the obese ADSC. We determined the senescence-associated (SA) β-galactosidase activity by visualizing it as a blue stain in cells. PKCδVIII over-expressing lean ADSC showed lower senescence compared to control lean ADSC. Obese ADSC showed lower senescence compared to control lean ADSC as seen in Figure 5A-D.
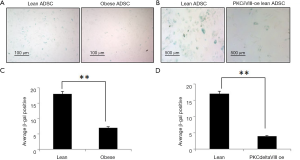
Discussion
In white adipose tissue (WAT) about 10% of adipocytes are renewed yearly (8,9). Adipose stem cells residing in the stromal vascular region initiate adipogenesis by proliferation of preadipocytes and subsequent differentiation into mature adipocytes. This process can be followed in vitro by isolating primary human ADSC from adipose tissue. We have characterized the stem cell markers using real time qPCR and flow cytometry in ADSC from an obese patient as well as from dedifferentiated fat (DFAT) cells (1). We further demonstrated the multipotency of ADSC by differentiating them in vitro into adipocytes and osteoblasts.
There are several factors which affect telomerase activity and senescence in ADSC. Increased expression of α+/β+ contributes to increased telomerase activity but is not solely responsible for increased telomere length. There are reports indicating that telomerase activity alone and not the components hTERT and hTR levels, regulate telomere length (10). Another possibility is that phosphorylation of hTERT increases telomerase activity. PKCα, c-Abl and Akt kinase (11-13) are implicated in hTERT phosphorylation. Alternatively spliced variants of hTERT are reported in several cancers (14,15) as well as during human development (5). Here, we report increase in α+/β+ alternatively spliced variant in obesity.
Protein kinase C δ (PKCδ) is a serine/threonine kinase with roles in proliferation and apoptosis. We identified the human splice variant PKCδVIII and showed that it promoted survival in cells (6,16). During adipogenesis, expression of PKCδVIII increases as the preadipocytes are committed to differentiation to adipocytes. By day 4 (terminally differentiated) they exhibit lipid accumulation which is a hallmark of maturing adipocytes. Our results indicated that PKCδVIII reached peak expression by day 4 and its levels were maintained in mature adipocytes. Previously, we demonstrated that in obese preadipocytes, adipogenesis is dysregulated. Further, expression of PKCδVIII is high in obese ADSC even at day 0 before differentiation is initiated. PKCδVIII levels peak by day 2 and are maintained in mature adipocytes (2).
ADSC obese subjects express hTERT α+/β+ alternatively spliced variant; lean ADSC show very low expression and the other splice variants are not significantly different between lean and obese (unpublished observation, Patel lab). Inclusion of the α region and the β region on hTERT pre-mRNA during splicing generates the full-length α+/β+ splice variant. Additional splice variants that are reported show deletion of α or β or both α and β regions (17). In addition to this, exclusion of exon 2 is reported which produces a splice variant which results in a truncated protein (18). The roles of these splice variants are still being investigated but are thought to either act as dominant-negative inhibitor of telomerase activity or affect other extra-telomeric functions of TERT such as Wnt signaling or mitochondrial localization (19-21).
There are extensive studies performed on telomerase and hTERT in several cancers; however, this is the first study showing that full length hTERT splice variant expression is increased in obesity. Alternative splicing provides for regulation of gene expression as well as the ability to change in accordance to the changing environment and disease states. PKCδ is also alternatively spliced and here we show that the alternatively splice variant PKCδVIII increases expression of α+/β+ hTERT splice variant and telomerase protein expression in ADSC. Our results also indicate that PKCδVIII is not entirely responsible for the higher telomerase and hTERT expression seen in obese ADSC. This may be explained considering the additional factors affecting telomerase expression and activity as discussed above.
Obesity presents with additional complications of inflammation and cell death alongside hyperplasia and hypertrophy. Obese adipocytes secrete different levels of cytokines and adipokines. We show decreased senescence in obese ADSC; however mature adipocytes may show increased senescence due to stress and inflammation. Our results point to epigenetic modifications in obese ADSC which could influence the factors and hormones secreted by the obese adipocyte thereby changing its autocrine and paracrine functions.
Acknowledgements
We thank James Watson for help with establishing ADSC in culture.
Funding: This work was supported by the Department of Veterans Affairs Medical Research grant (NA Patel).
Footnote
Conflicts of Interest: The authors have no conflicts of interest to declare.
Disclaimer: The contents do not represent the views of the Department of Veterans Affairs or the United States Government.
References
- Watson JE, Patel NA, Carter G, et al. Comparison of Markers and Functional Attributes of Human Adipose-Derived Stem Cells and Dedifferentiated Adipocyte Cells from Subcutaneous Fat of an Obese Diabetic Donor. Advances in Wound Care 2014;3:219-28. [PubMed]
- Carter G, Apostolatos A, Patel R, et al. Dysregulated Alternative Splicing Pattern of PKC during Differentiation of Human Preadipocytes Represents Distinct Differences between Lean and Obese Adipocytes. ISRN Obes 2013;2013:161345.
- Blackburn EH. Telomere states and cell fates. Nature 2000;408:53-6. [PubMed]
- Ulaner GA, Hu JF, Vu TH, et al. Telomerase activity in human development is regulated by human telomerase reverse transcriptase (hTERT) transcription and by alternate splicing of hTERT transcripts. Cancer Res 1998;58:4168-72. [PubMed]
- Ulaner GA, Hu JF, Vu TH, et al. Tissue-specific alternate splicing of human telomerase reverse transcriptase (hTERT) influences telomere lengths during human development. Int J Cancer 2001;91:644-9. [PubMed]
- Jiang K, Apostolatos AH, Ghansah T, et al. Identification of a novel antiapoptotic human protein kinase C delta isoform, PKCdeltaVIII in NT2 cells. Biochemistry 2008;47:787-97. [PubMed]
- Katakura Y, Udono M, Katsuki K, et al. Protein kinase C delta plays a key role in cellular senescence programs of human normal diploid cells. J Biochem 2009;146:87-93. [PubMed]
- Arner P, Spalding KL. Fat cell turnover in humans. Biochem Biophys Res Commun 2010;396:101-4. [PubMed]
- Spalding KL, Arner E, Westermark PO, et al. Dynamics of fat cell turnover in humans. Nature 2008;453:783-7. [PubMed]
- Swiggers SJ, Nibbeling HA, Zeilemaker A, et al. Telomerase activity level, but not hTERT mRNA and hTR level, regulates telomere length in telomerase-reconstituted primary fibroblasts. Exp Cell Res 2004;297:434-43. [PubMed]
- Kharbanda S, Kumar V, Dhar S, et al. Regulation of the hTERT telomerase catalytic subunit by the c-Abl tyrosine kinase. Curr Biol 2000;10:568-75. [PubMed]
- Chang JT, Lu YC, Chen YJ, et al. hTERT phosphorylation by PKC is essential for telomerase holoprotein integrity and enzyme activity in head neck cancer cells. Br J Cancer 2006;94:870-8. [PubMed]
- Kimura A, Ohmichi M, Kawagoe J, et al. Induction of hTERT expression and phosphorylation by estrogen via Akt cascade in human ovarian cancer cell lines. Oncogene 2004;23:4505-15. [PubMed]
- Rha SY, Jeung HC, Park KH, et al. Changes of telomerase activity by alternative splicing of full-length and beta variants of hTERT in breast cancer patients. Oncol Res 2009;18:213-20. [PubMed]
- Brambilla C, Folini M, Gandellini P, et al. Oligomer-mediated modulation of hTERT alternative splicing induces telomerase inhibition and cell growth decline in human prostate cancer cells. Cell Mol Life Sci 2004;61:1764-74. [PubMed]
- Apostolatos H, Apostolatos A, Vickers T, et al. Vitamin A metabolite, all-trans-retinoic acid, mediates alternative splicing of protein kinase C deltaVIII (PKCdeltaVIII) isoform via splicing factor SC35. J Biol Chem 2010;285:25987-95. [PubMed]
- Lincz LF, Mudge LM, Scorgie FE, et al. Quantification of hTERT splice variants in melanoma by SYBR green real-time polymerase chain reaction indicates a negative regulatory role for the beta deletion variant. Neoplasia 2008;10:1131-7. [PubMed]
- Withers JB, Ashvetiya T, Beemon KL. Exclusion of exon 2 is a common mRNA splice variant of primate telomerase reverse transcriptases. PLoS One 2012;7:e48016. [PubMed]
- Maida Y, Yasukawa M, Furuuchi M, et al. An RNA-dependent RNA polymerase formed by TERT and the RMRP RNA. Nature 2009;461:230-5. [PubMed]
- Palma M, Parker A, Hojjat-Farsangi M, et al. Telomere length and expression of human telomerase reverse transcriptase splice variants in chronic lymphocytic leukemia. Exp Hematol 2013;41:615-26. [PubMed]
- Park JI, Venteicher AS, Hong JY, et al. Telomerase modulates Wnt signalling by association with target gene chromatin. Nature 2009;460:66-72. [PubMed]
Cite this article as: Carter G, Patel R, Apostolatos A, Murr M, Cooper DR, Patel NA. Protein kinase C delta (PKCδ) splice variant modulates senescence via hTERT in adipose-derived stem cells. Stem Cell Investig 2014;1:3.