Interplay of retinal determination gene network with TGF-β signaling pathway in epithelial-mesenchymal transition
Introduction
Recent studies demonstrated that members of the Retinal Determination Gene Network (RDGN), a key gene network in organ development, have been shown to serve as co-regulators of transforming growth factors β (TGF-β) signaling thereby regulating cancer development (1,2). TGF-β signal transducers to modify TGF-β signal in tumor initiation and progression are well documented with various cancers (3). TGF-β and bone morphogenetic proteins (BMPs) are members of the TGF-β superfamily, which contains over 30 ligands that can be categorized into subgroups: Activin, Nodal, BMPs, growth and differentiation factors (GDFs), Anti-müllerian hormone (AMH) and TGF-βs (4). Studies in the past few decades have demonstrated that TGF-β not only plays an essential role in the control of cell proliferation, tissue differentiation and embryonic development but also influences stem cell behaviors (3). Other signaling components have been shown to interact with TGF-β (5). The interplay between RDGN with TGF-β/BMP signaling to influence epithelial-mesenchymal transition (EMT) and cancer stem cell fate would be a tremendous opportunity for developing novel strategies for targeted therapies.
TGF-β/BMP signaling
TGF-β is a ubiquitous cytokine with profound effects on epithelial and other tissues, and also well known for its functions in the regulation of cell fate specification, compartment boundary establishment as well as cell proliferation and death (3). Two fundamental types of serine/threonine kinase transmembrane receptors are responsible for amplifying the signal of TGF-β superfamily ligands in human genome, type I and type II. For some ligands, additional co-receptors are necessary for optimal ligand binding to the type I-type II receptor complex. After a constitutive activation of the receptor complex, the type II receptor recruits and phosphorylates the type I receptor on several serines and threonines, which in the context provide a binding site for the downstream substrates, the receptor-regulator SMADs (R-SMADs) (6). SMAD members could shuttle between the cytoplasm and nucleus. Upon phosphorylation, the R-SMADs complex accumulates in the nucleus, then associated with other transcription factors such as FAST1 and FAST2, which recruit SMADs complex to specific DNA sequences (7). Regulation of SMADs complex occurs at a number of different levels, either positively through abundance of coactivators of the p300/CREB-binding protein class (8), or negatively by recruiting the Sno/Ski family (9,10). During carcinogenesis, the normal hierarchical organization of the biological microenvironment breaks down, at least in a significant part, owing to misexpression in TGF-β/Smads family (Figure 1).
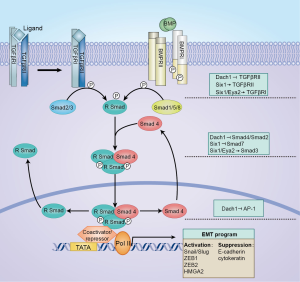
Similar to TGF-β pathway, the BMP signaling cascade is composed of two types of receptor serine/threonine protein kinases known as type I and type II receptors, and transcription factors belonging to the SMAD family which transduce the BMP signal to the nucleus (5). Specifically, binding of BMP ligands to the type II receptor leads to phosphorylation of the type I receptor, which in turn activates the BMP R-Smads, Smads 1/5/8. Phosphorylated R-SMADs form heterodimers with SMAD4 (a common co-SMAD for the BMP as well as the TGF-β signaling pathway), and translocate into the nucleus, in which they regulate transcription of the BMP downstream target genes. In contrast to the Smad-dependent canonical pathway, the emerging evidence points to a role for SMAD independent fashion via TAK1, MKK3 and p38 MAPK in BMP signaling (11).
Indeed, TGF-β pathway displays a dual role in cancer development, acting as a tumor suppressor in early lesions but enhancing successful metastasis in the subset of advanced tumors (12,13). TGF-β induces EMT majorly through transcriptional activation of mesenchymal genes, such as Snail/Slug, Zeb1, Twist, and repression of epithelial genes, like E-cadherin and cytokeratin (14) (Figure 1). Post-transcriptional regulation by TGF-β also mediated EMT process, such as translation of Snail and splicing of epithelial splicing regulatory protein 1 (ESRP) (15). The reprogramming of oncogenic gene expression during EMT progression could be induced and controlled by TGF-β signaling pathway (16). Because TGF-β signaling is critical for EMT during morphogenesis, and cancer cells with EMT acquire enhanced migration and invasion in a tumorigenic context (17), it is likely that the ability of TGF-β to promote metastasis is at least in part due to its ability to induce an EMT. Interestingly, mesenchymal stromal cells secrete TGF-β to drive EMT of cancer cell (18). On the other hand, cancer-associated fibroblasts activate TGF-β signaling to maintain stemness phenotype of cancer cell (19).
RDGN signal network
The RDGN, which functions as an administrator in drosophila eye specification, has been proved to play key roles in the formation of many different organs as well as tumorigenesis. This regulatory framework is mainly consisted of dachshund (dac/Dach), eyes absent (eya/Eya) and sin oculis (so/Six) etc. (20,21). As a crucial member of RDGN network, the dac gene was initially cloned as a dominant inhibitor of ellipse in drosophila, whereas the DACH1 may serve as a novel tumor suppressor though its inhibition on malignant proliferation as well as migration and invasion in cultured cells (22,23). Several lines of evidence suggest that DACH1 regulates expression of target genes either through interacting with DNA-binding transcription factors (c-Jun, Smads, Six, and ER) (1,23-25) or direct association with chromatin DNA (26,27). For example, DACH1 combines with AP-1 family to inhibit breast and renal cancer cell proliferation (28,29). DACH1 attenuates oncogenic FoxC1 by competition for forkhead binding sites (26). Recent studies highlight the significance of DACH1 in the regulation of the tumor microenvironment. Not only transcriptionally suppresses the interleukin (IL) -8, DACH1 is but also shown to be an endogenous inhibitor of CXCL signaling to restraint cytokine abundance during cell growth and migration (30-32).
Members of Six family, a mammalian homolog of the Drosophila sine oculis gene, belong to the homebox genes and are conserved during the evolution of species (33). Traditionally, Six coordinates with Eya as an active complex in tissue specification like eye, kidney and muscle (25). Disruption of the SIX-EYA complex gives rise to the branchiootorenal (BOR) syndrome in human, an autosomal dominant genetic disorder involving kidneys, ears and neck (34). During the early stage, Six1 promotes the progenitor cell proliferation and survival through activation or repression of a diverse range of downstream target genes. Once the organ development is completed, the expression of Six1 keeps low or undetectable in adult tissue maintenance (35). However, reactivations of SIX1 by unknown mechanism are reported in various types of cancer (36). Ectopic expression of Six1 could perturb the normal self-renewal system, which contributes to tumor onset and strongly correlates with tumor aggressiveness (37). Indeed, SIX1 accelerates the cell cycle process depending on either cyclin D1 or cyclin A1 abundance, and increases cell motility and invasiveness (38,39). On the other hand, knockdown of SIX1 in animal models would cause a drastic reduction in tumor size and metastasis, most of which relies on the inactivation of Wnt signaling (40). Thus, inhibition of SIX1 function would be therapeutically relevant in many different cancers.
A prominent role of mammalian eye absent (EYA) proteins is tantamount to drive cell-fate determination in a broad spectrum of cells and tissues (41). As a transcriptional co-activator to Six family, Eya is recruited in the context of local chromatin and release the suppressive status of Six to permit target gene expression (25). Besides, phosphatase activity is another instinct feature of Eya family. The evidences in the DNA damage as well as the innate immune response emphasize the importance of Eya phosphatase function in the dynamic processes of organization (42,43). Moreover, EYA2 dephosphorylates of residue in Y36 on estrogen receptor β (ERβ) to modify its the suppressive role in tumor development and dissemination (44). It is becoming increasingly clear that aberrant expression of EYA proteins can induce an oncogenic activity in breast and ovarian cancers (45-47). In particular, EYA2 is shown to promote proliferation, migration, and invasion of breast cancer cells. In this respect, a class of Eya phosphatase inhibitors identified by Dr. Ford’s group offers a therapeutic opportunity by targeting Eya2 overexpression tumor (48).
Integration of TGF-β/BMP signaling with RDGNs in EMT regulation
The significance of RDGN in the interplay with TGF-β signaling pathways appears in parallel with an understanding of each key regulator’s biochemistry and its contribution to tumor process. This network can be approximately classified into two types depending on the final effecting in the signaling. The first class is defined as a repressor of TGF-β pathway. For example, dac combines with dpp to function as a tight regulatory loop, which is responsible for maintaining the correct formation of legs and eyes in Drosophila (49). During chicken developmental processes, Dach1 interacts with Smads to make a large repressor complex, illuminating its role as an intracellular modulator of BMP signaling (50). DACH1 degrades TGF-β induction of activator protein-1 (AP-1) and represses SMAD signaling in gene reporter assays and endogenous TGF-β responsive genes by microarray analysis (1). Further molecular analysis shows the negative regulation of TGF-β by DACH1 requires the DACH1 DS domain, since this structure recruits NCoR to bind SMAD4-binding site (1,51). Subsequent analysis identified that DACH1 shared structural homology to Ski/Sno pro-oncogenes, both of which repress AP-1 and Smad signaling associate with TGF-β (52). In ovary cancer, high levels of DACH1 correlate with adverse tendency of expression in TGFβRII and Smad4, providing another evidence of mutual regulation (2). Meanwhile, overexpression of DACH1 allows a lower level of phosphorylated Smad2 in colorectal cancer cells as well as in gastric cancer, in which DACH1 in part perturbs TGF-β/Smad pathway induced EMT during tumorigenesis (51,53). Of note, the inverse relationship between DACH1 and BMPs is also reported in other models (54,55), extending potential mechanism for the communication between DACH1 and TGF-β/BMP superfamily.
The second class of RDGNs is characterized by remarkable activation by Eya and Six in TGF-β signaling during metastases. Especially, as a chief member of Six family, Six1 participates in the regulation of TGF-β at multiple levels through diverse mechanisms. Evidence is mounting that Six1 expression has the potential to selectively promote the pro-metastatic activity of TGF-β while antagonizing its growth-inhibitory function, which is in conjunction with nuclear accumulation of Smad3. Inhibition of the TGF-β signaling could reverse element of Six1-induced EMT, which was interpreted as the hints that Six1-induced EMT requires TGF-β signal (56). Intriguingly, several lines of evidence indicate that Six1 could be the crucial mediator in switching TGF-β signaling from suppressive performance to tumor promotional. Indeed, Six1 homeoprotein downstream targets are involved in TGF-β pathway, such as the up-regulation of TGFβRI and TGFβRII by Six1 significantly contributes to the activation of TGF-β signal (57,58). Although upregulation of TGFβRI in MCF7 cells is sufficient to induce TGF-β signaling and EMT, it is clear not sufficient to increase metastasis in a tumorigenic context (57), suggesting other underlying downstream targets coordinated with TGFβR to reinforce the activation induced by Six1. An elegant study from Anna et al. demonstrates a cluster of miRNA-106b-25 upregulated by Six1 homeoprotein has physiologically relevant substrate of Smad7, recognized as a dominant inhibitor in Smad family, and also activate the TGF-β pathways, providing a novel mechanism in understanding the switch behavior of Six1 in TGF-β signaling from tumor suppressive to tumor promotional (59). Intriguingly, the Six1 mediated increasing of TGFβRI and Smad3 as well as the induced profiles of EMT and cancer stem cell can be reversed in the absence of Eya2, raising the possibility that Eya family is necessary for the pro-tumorigenic functions of Six1 to active TGF-β signaling (46).
Other mechanism involved in the regulation of EMT by RDGNs
To date, great endeavor has been applied to explore other molecular underpinnings of RDGN members in the regulation of EMT progress. Besides coordination with TGF-β superfamily, ectopic expression of DACH/EYA/SIX genes could respond to other oncogenic signaling to maintain the disseminated tumor cells. As DACH1 can function as oppose inducer of EMT, loss of DACH1 in tumor would permit the development of a migratory, invasive cell state. By inactivation of the YB-1 mediated induction of Snail translation, recovery the expression of DACH1 reduces the number of mammospheres, and even reverses the EMT in basal-like forms of breast cancer, a most aggressive subtype (60). Besides, DACH1 directly suppresses the transcriptional activity of SNAI1 to increase the expression of E-cadherin in breast cancer cell lines, limiting the ability to migration and invasion (61,62). Another mechanism of DACH1’s ability to repress EMT might come from the inactivation of Wnt/β-catenin signaling, since our group recently finds that β-catenin is a novel target of DACH1 and overexpression of DACH1 gives rise to phosphorylation of GSK3β (53). Besides, DACH1 is capable of reversing the H-Ras and c-Myc induced oncogenic phenotype and diminishes the magnificent proliferation of breast cancer (32).
Standing on the other side of RD genetic network is EYA/SIX. In breast and cervical cancer, SIX1 and VEGF-C consist of a prometastatic axis and function in peritumoral and intratumoral lymphangiogenesis, lymphatic invasion and distant metastasis during EMT development (63,64). Moreover, the subpopulation of cancer stem cell in mammary gland plays a critical role for driving Six1-mediated tumorigenesis, which in part relies on the activation on Wnt signaling (40). Six1 enhances a tumor initiating phenotype in part through MEK/ERK signaling, another crucial pathway in maintenance the proper stem cell properties (65). Additional mechanisms of SIX1 regulating EMT include repression of miR-200-family expression, resulting in posttranscriptional activation of ZEB1 to ignite carcinogenesis and promote EMT (66). Although the individual value of EYA family in EMT progress is currently unknown, the tight interaction of EYA-SIX as a transcriptional complex cannot rule out the possibilities that Eya participates in every step of EMT regulation induced by Six family.
Conclusions
The understanding of the molecular mechanism involved in tumor cell dissemination is the key to identify potential therapeutic targets to prevent or limit the extent of metastasis. As a critical mechanism of migration during development, EMT program could be hijacked in cancer cell by abnormal activation of developmental pathways, like TGF-β P signaling. The integration of TGF-β P pathway with the main members in RDGNs, DACH/EYA/SIX, would provide novel insight in the regulation of EMT. It is well known that cancer cells with EMT phenotypes acquire cancer stem cell properties and gain therapeutic resistance. If modification of RDGN-dependent TGF-β signaling can reverse the EMT phenotype is worthy further study. Reinstalling the normal hierarch of this signaling pathway by targeting DACH/EYA/SIX may offer us an opportunity to block oncogenic EMT process.
Acknowledgements
Funding: This work was supported from the National Science Foundation of China (Grant No. 81172422, 81261120395, 81072169).
Footnote
Conflicts of Interest: The authors have no conflicts of interest to declare.
References
- Wu K, Yang Y, Wang C, et al. DACH1 inhibits transforming growth factor-beta signaling through binding Smad4. J Biol Chem 2003;278:51673-84. [PubMed]
- Sunde JS, Donninger H, Wu K, et al. Expression profiling identifies altered expression of genes that contribute to the inhibition of transforming growth factor-beta signaling in ovarian cancer. Cancer Res 2006;66:8404-12. [PubMed]
- Massagué J. TGFbeta in Cancer. Cell 2008;134:215-30. [PubMed]
- Wakefield LM, Hill CS. Beyond TGFβ: roles of other TGFβ superfamily members in cancer. Nat Rev Cancer 2013;13:328-41. [PubMed]
- Massagué J, Chen YG. Controlling TGF-beta signaling. Genes Dev 2000;14:627-44. [PubMed]
- Shi Y, Massagué J. Mechanisms of TGF-beta signaling from cell membrane to the nucleus. Cell 2003;113:685-700. [PubMed]
- Chen X, Rubock MJ, Whitman M. A transcriptional partner for MAD proteins in TGF-beta signalling. Nature 1996;383:691-6. [PubMed]
- Janknecht R, Wells NJ, Hunter T. TGF-beta-stimulated cooperation of smad proteins with the coactivators CBP/p300. Genes Dev 1998;12:2114-9. [PubMed]
- Sun Y, Liu X, Eaton EN, et al. Interaction of the Ski oncoprotein with Smad3 regulates TGF-beta signaling. Mol Cell 1999;4:499-509. [PubMed]
- Stroschein SL, Wang W, Zhou S, et al. Negative feedback regulation of TGF-beta signaling by the SnoN oncoprotein. Science 1999;286:771-4. [PubMed]
- Kim SI, Kwak JH, Zachariah M, et al. TGF-beta-activated kinase 1 and TAK1-binding protein 1 cooperate to mediate TGF-beta1-induced MKK3-p38 MAPK activation and stimulation of type I collagen. Am J Physiol Renal Physiol 2007;292:F1471-8. [PubMed]
- Chen CR, Kang Y, Massagué J. Defective repression of c-myc in breast cancer cells: A loss at the core of the transforming growth factor beta growth arrest program. Proc Natl Acad Sci U S A 2001;98:992-9. [PubMed]
- Siegel PM, Massagué J. Cytostatic and apoptotic actions of TGF-beta in homeostasis and cancer. Nat Rev Cancer 2003;3:807-21. [PubMed]
- Saitoh M, Miyazawa K. Transcriptional and post-transcriptional regulation in TGF-β-mediated epithelial-mesenchymal transition. J Biochem 2012;151:563-71. [PubMed]
- Saitoh M. Epithelial-mesenchymal transition is regulated at post-transcriptional levels by transforming growth factor-β signaling during tumor progression. Cancer Sci 2015;106:481-8. [PubMed]
- Zavadil J, Böttinger EP. TGF-beta and epithelial-to-mesenchymal transitions. Oncogene 2005;24:5764-74. [PubMed]
- Katsuno Y, Lamouille S, Derynck R. TGF-β signaling and epithelial-mesenchymal transition in cancer progression. Curr Opin Oncol 2013;25:76-84. [PubMed]
- Mele V, Muraro MG, Calabrese D, et al. Mesenchymal stromal cells induce epithelial-to-mesenchymal transition in human colorectal cancer cells through the expression of surface-bound TGF-β. Int J Cancer 2014;134:2583-94. [PubMed]
- Hasegawa T, Yashiro M, Nishii T, et al. Cancer-associated fibroblasts might sustain the stemness of scirrhous gastric cancer cells via transforming growth factor-β signaling. Int J Cancer 2014;134:1785-95. [PubMed]
- Liu Y, Han N, Zhou S, et al. The DACH/EYA/SIX gene network and its role in tumor initiation and progression. Int J Cancer 2015. [Epub ahead of print].
- Pignoni F, Hu B, Zavitz KH, et al. The eye-specification proteins So and Eya form a complex and regulate multiple steps in Drosophila eye development. Cell 1997;91:881-91. [PubMed]
- Mardon G, Solomon NM, Rubin GM. dachshund encodes a nuclear protein required for normal eye and leg development in Drosophila. Development 1994;120:3473-86. [PubMed]
- Popov VM, Zhou J, Shirley LA, et al. The cell fate determination factor DACH1 is expressed in estrogen receptor-alpha-positive breast cancer and represses estrogen receptor-alpha signaling. Cancer Res 2009;69:5752-60. [PubMed]
- Wu K, Liu M, Li A, et al. Cell fate determination factor DACH1 inhibits c-Jun-induced contact-independent growth. Mol Biol Cell 2007;18:755-67. [PubMed]
- Li X, Oghi KA, Zhang J, et al. Eya protein phosphatase activity regulates Six1-Dach-Eya transcriptional effects in mammalian organogenesis. Nature 2003;426:247-54. [PubMed]
- Zhou J, Wang C, Wang Z, et al. Attenuation of Forkhead signaling by the retinal determination factor DACH1. Proc Natl Acad Sci U S A 2010;107:6864-9. [PubMed]
- Zhou J, Liu Y, Zhang W, et al. Transcription elongation regulator 1 is a co-integrator of the cell fate determination factor Dachshund homolog 1. J Biol Chem 2010;285:40342-50. [PubMed]
- Chu Q, Han N, Yuan X, et al. DACH1 inhibits cyclin D1 expression, cellular proliferation and tumor growth of renal cancer cells. J Hematol Oncol 2014;7:73. [PubMed]
- Wu K, Li A, Rao M, et al. DACH1 is a cell fate determination factor that inhibits cyclin D1 and breast tumor growth. Mol Cell Biol 2006;26:7116-29. [PubMed]
- Chen K, Wu K, Jiao X, et al. The Endogenous Cell-Fate Factor Dachshund Restrains Prostate Epithelial Cell Migration via Repression of Cytokine Secretion via a CXCL Signaling Module. Cancer Res 2015;75:1992-2004. [PubMed]
- Han N, Yuan X, Wu H, et al. DACH1 inhibits lung adenocarcinoma invasion and tumor growth by repressing CXCL5 signaling. Oncotarget 2015;6:5877-88. [PubMed]
- Wu K, Katiyar S, Li A, et al. Dachshund inhibits oncogene-induced breast cancer cellular migration and invasion through suppression of interleukin-8. Proc Natl Acad Sci U S A 2008;105:6924-9. [PubMed]
- Wu W, Ren Z, Li P, et al. Six1: a critical transcription factor in tumorigenesis. Int J Cancer 2015;136:1245-53. [PubMed]
- Patrick AN, Cabrera JH, Smith AL, et al. Structure-function analyses of the human SIX1-EYA2 complex reveal insights into metastasis and BOR syndrome. Nat Struct Mol Biol 2013;20:447-53. [PubMed]
- Zou D, Silvius D, Fritzsch B, et al. Eya1 and Six1 are essential for early steps of sensory neurogenesis in mammalian cranial placodes. Development 2004;131:5561-72. [PubMed]
- Blevins MA, Towers CG, Patrick AN, et al. The SIX1-EYA transcriptional complex as a therapeutic target in cancer. Expert Opin Ther Targets 2015;19:213-25. [PubMed]
- Christensen KL, Patrick AN, McCoy EL, et al. The six family of homeobox genes in development and cancer. Adv Cancer Res 2008;101:93-126. [PubMed]
- Yu Y, Davicioni E, Triche TJ, et al. The homeoprotein six1 transcriptionally activates multiple protumorigenic genes but requires ezrin to promote metastasis. Cancer Res 2006;66:1982-9. [PubMed]
- Coletta RD, Christensen KL, Micalizzi DS, et al. Six1 overexpression in mammary cells induces genomic instability and is sufficient for malignant transformation. Cancer Res 2008;68:2204-13. [PubMed]
- McCoy EL, Iwanaga R, Jedlicka P, et al. Six1 expands the mouse mammary epithelial stem/progenitor cell pool and induces mammary tumors that undergo epithelial-mesenchymal transition. J Clin Invest 2009;119:2663-77. [PubMed]
- Jemc J, Rebay I. The eyes absent family of phosphotyrosine phosphatases: properties and roles in developmental regulation of transcription. Annu Rev Biochem 2007;76:513-38. [PubMed]
- Cook PJ, Ju BG, Telese F, et al. Tyrosine dephosphorylation of H2AX modulates apoptosis and survival decisions. Nature 2009;458:591-6. [PubMed]
- Okabe Y, Sano T, Nagata S. Regulation of the innate immune response by threonine-phosphatase of Eyes absent. Nature 2009;460:520-4. [PubMed]
- Yuan B, Cheng L, Chiang HC, et al. A phosphotyrosine switch determines the antitumor activity of ERβ. J Clin Invest 2014;124:3378-90. [PubMed]
- Wu K, Li Z, Cai S, et al. EYA1 phosphatase function is essential to drive breast cancer cell proliferation through cyclin D1. Cancer Res 2013;73:4488-99. [PubMed]
- Farabaugh SM, Micalizzi DS, Jedlicka P, et al. Eya2 is required to mediate the pro-metastatic functions of Six1 via the induction of TGF-β signaling, epithelial-mesenchymal transition, and cancer stem cell properties. Oncogene 2012;31:552-62. [PubMed]
- Zhang L, Yang N, Huang J, et al. Transcriptional coactivator Drosophila eyes absent homologue 2 is up-regulated in epithelial ovarian cancer and promotes tumor growth. Cancer Res 2005;65:925-32. [PubMed]
- Krueger AB, Drasin DJ, Lea WA, et al. Allosteric inhibitors of the Eya2 phosphatase are selective and inhibit Eya2-mediated cell migration. J Biol Chem 2014;289:16349-61. [PubMed]
- González-Crespo S, Abu-Shaar M, Torres M, et al. Antagonism between extradenticle function and Hedgehog signalling in the developing limb. Nature 1998;394:196-200. [PubMed]
- Kida Y, Maeda Y, Shiraishi T, et al. Chick Dach1 interacts with the Smad complex and Sin3a to control AER formation and limb development along the proximodistal axis. Development 2004;131:4179-87. [PubMed]
- Yan W, Wu K, Herman JG, et al. Epigenetic silencing of DACH1 induces the invasion and metastasis of gastric cancer by activating TGF-β signalling. J Cell Mol Med 2014;18:2499-511. [PubMed]
- Wilson JJ, Malakhova M, Zhang R, et al. Crystal structure of the dachshund homology domain of human SKI. Structure 2004;12:785-92. [PubMed]
- Yan W, Wu K, Herman JG, et al. Epigenetic regulation of DACH1, a novel Wnt signaling component in colorectal cancer. Epigenetics 2013;8:1373-83. [PubMed]
- Kumagai T, Tomari K, Shimizu T, et al. Alteration of gene expression in response to bone morphogenetic protein-2 in androgen-dependent human prostate cancer LNCaP cells. Int J Mol Med 2006;17:285-91. [PubMed]
- Schild R, Knüppel T, Konrad M, et al. Double homozygous missense mutations in DACH1 and BMP4 in a patient with bilateral cystic renal dysplasia. Nephrol Dial Transplant 2013;28:227-32. [PubMed]
- Micalizzi DS, Christensen KL, Jedlicka P, et al. The Six1 homeoprotein induces human mammary carcinoma cells to undergo epithelial-mesenchymal transition and metastasis in mice through increasing TGF-beta signaling. J Clin Invest 2009;119:2678-90. [PubMed]
- Micalizzi DS, Wang CA, Farabaugh SM, et al. Homeoprotein Six1 increases TGF-beta type I receptor and converts TGF-beta signaling from suppressive to supportive for tumor growth. Cancer Res 2010;70:10371-80. [PubMed]
- Xu H, Zhang Y, Altomare D, et al. Six1 promotes epithelial-mesenchymal transition and malignant conversion in human papillomavirus type 16-immortalized human keratinocytes. Carcinogenesis 2014;35:1379-88. [PubMed]
- Smith AL, Iwanaga R, Drasin DJ, et al. The miR-106b-25 cluster targets Smad7, activates TGF-β signaling, and induces EMT and tumor initiating cell characteristics downstream of Six1 in human breast cancer. Oncogene 2012;31:5162-71. [PubMed]
- Wu K, Chen K, Wang C, et al. Cell fate factor DACH1 represses YB-1-mediated oncogenic transcription and translation. Cancer Res 2014;74:829-39. [PubMed]
- Zhao F, Wang M, Li S, et al. DACH1 inhibits SNAI1-mediated epithelial-mesenchymal transition and represses breast carcinoma metastasis. Oncogenesis 2015;4:e143. [PubMed]
- Liu Y, Zhou R, Yuan X, et al. DACH1 is a novel predictive and prognostic biomarker in hepatocellular carcinoma as a negative regulator of Wnt/β-catenin signaling. Oncotarget 2015;6:8621-34. [PubMed]
- Wang CA, Jedlicka P, Patrick AN, et al. SIX1 induces lymphangiogenesis and metastasis via upregulation of VEGF-C in mouse models of breast cancer. J Clin Invest 2012;122:1895-906. [PubMed]
- Liu D, Li L, Zhang XX, et al. SIX1 promotes tumor lymphangiogenesis by coordinating TGFβ signals that increase expression of VEGF-C. Cancer Res 2014;74:5597-607. [PubMed]
- Iwanaga R, Wang CA, Micalizzi DS, et al. Expression of Six1 in luminal breast cancers predicts poor prognosis and promotes increases in tumor initiating cells by activation of extracellular signal-regulated kinase and transforming growth factor-beta signaling pathways. Breast Cancer Res 2012;14:R100. [PubMed]
- Ono H, Imoto I, Kozaki K, et al. SIX1 promotes epithelial-mesenchymal transition in colorectal cancer through ZEB1 activation. Oncogene 2012;31:4923-34. [PubMed]
Cite this article as: Liu Y, Kong D, Wu H, Yuan X, Xu H, Zhang C, Wu G, Wu K. Interplay of retinal determination gene network with TGF-β signaling pathway in epithelial-mesenchymal transition. Stem Cell Investig 2015;2:12.