Current insights into the diagnosis and treatment of inherited bone marrow failure syndromes in China
Bone marrow failure syndromes (BMFs) are a group of heterogeneous disorders characterized by one- or two-lineage cytopenia or pancytopenia caused by inefficient hematopoiesis in the bone marrow (1-3). The current incidences of BMFs in Europe and North America are approximately 2/100,000, which is slightly lesser than some local American study findings and previous European epidemiological investigations (2). BMFs morbidity in Asia is 4 to 7 per 100,000, but there are no accurate statistics on the morbidity among children and adolescents in China. There are two subgroups of pediatric BMFs, acquired BMFs (ABMFs) and inherited BMFs (IBMFs). ABMFs account for 80% of pediatric BMFs. Although guidelines for the diagnosis and treatments of IBMFs have been recently published (4), improvements are still needed in making accurate diagnosis and suitable treatment of pediatric patients with IBMFs.
Clinical characteristics and pathogenesis of IBMFs
The classification of IBMFs
IBMFs include Fanconi anemia (FA), dyskeratosis congenita (DC), Shwachman-Diamond syndrome (SDS), Diamond-Blackfan anemia (DBA), congenital amegakaryocytic thrombocytopenia (CAMT), thrombocytopenia absent radii (TAR), and severe congenital neutropenia (SCN) or Kostmann neutropenia (5-8). In addition, patients with bone marrow failure who cannot be classified into the above subtypes are considered to have unclassified-IBMFs (9).
The common features of IBMFs
IBMFs are a rare heterogeneous group of hereditary disorders characterized by progressive single-lineage or multi-lineage cytopenia and a high risk for myelodysplasia (MDS) and acute myeloid leukemia (AML).
The typical clinical features are congenital physical malformations, including café-au-lait spots, microcephaly and radial ray abnormalities in FA patients; ectodermal dysplasia in DC patients; malformations involving the upper limbs, head, and urogenital or cardiovascular systems in DBA patients; and the absence of radii in TAR patients. However, numerous patients with IBMFs have atypical presentations or no overt physical abnormalities. The majority of patients are diagnosed at roughly seven years of age, although some patients are first diagnosed in adulthood. Notably, family histories of leukemia, solid tumors, birth defects, and other physical deformities often exist in these patients. The frequency of abnormalities in IBMFs is listed in Table 1 (10-13).
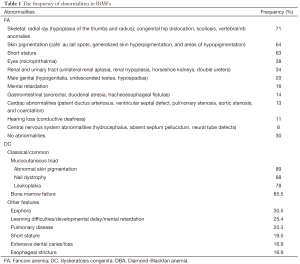
Full table
The immune status in pediatric patients with IBMFs
The recent largest series research showed that children with FA had lower B- and NK cells and normal immunoglobulins, total lymphocytes, and CD4 T-cells. Patients with DC had normal immunoglobulins but lower total lymphocytes and lower T-, B-, and NK-cell. Most patients with DBA and SDS had normal immunoglobulins and lymphocytes. Lymphoproliferative responses from phytohemagglutinin-stimulated cultures were similar across patient groups and controls. Only patients with severe BMF, particularly those with FA and DC, had higher serum G-CSF and Flt3-ligand and lower RANTES levels compared with all other groups or relatives (14).
The common pathophysiology of IBMFs
Current advances in the field of IBMFs have revealed diverse mutant genes. Many of these genes encode proteins that are involved in cellular housekeeping pathways, and perturbing these pathways can cause cellular senescence and apoptosis as well as predispose cells to malignant transformation (15). For example, in FA, the risk of developing solid tumors is potentially increased one thousand-fold among juveniles, and the average age of onset is 16 years, with squamous cell carcinoma and head and neck cancer being more common. Other solid tumors include cerebral myelocytomas and astrocytomas, esophageal cancer, vulvar cancer, gynecologic squamous cell carcinoma, liver cancer, Wilms tumor, and breast cancer. Two or more concurrent tumors that may be either hematologic or non-hematological observed in few patients. Moreover, papilloma virus infections responsible for the significantly increased incidence of the above mentioned tumors in FA patients, especially mucosa epithelial carcinoma (16). The mechanism of cancer progression with the background of increased apoptosis in patients with FA is unclear. We verified that the average relative telomere length in DC patients was remarkably shorter than those innormal children of the same age. Furthermore, we detected TINF2 c.811C > T(Q271X) and TINF2 c.848C > A(P283H) mutations in two DC patients for the first time in China, is indicating that early detection of related genes and telomere length measurements may help avoid misdiagnosis (17). Recent discoveries have revealed that Bmi1 expression in human hematopoietic stem and progenitor cells (HSPCs) in patients with DBA is correlated with the expression of certain ribosomal protein genes, suggesting that Bmi1 deficiency may play a pathological role in DBA and other ribosomopathies (18).
Currently, ribosomal pathway dysfunction has been associated with pathogenesis of IBMFs. Ribosomal proteins, SDBS and DKC are all involved in normal ribosome biogenesis and protein translation. In general, the heterozygous DBA mutations result in loss of function in a single copy of a ribosomal protein gene, causing disruption in ribosomal biogenesis, and decreased levels of protein synthesis and failing of translation of specific mRNAs (19-22). The known involved cell-signaling pathways include MDM2/HDM2-p53 and mTOR signaling. Mutations in RPL5 and RPL11 destroyed the formation of a pre-ribosomal complex that coordinately inhibits HDM2 to upregulate p53, finally causing increased level of P53 (23). Recent evidence showed that the L-leucine was effective in some DBA patients. The L-leucine may increase protein translation through activation of mTOR pathway (24-26). SBDS promotes the release of EIF6 from the pre-60S ribosome, which is required for the formation of a mature 80S functional ribosome (27-29). Approximately half of the genes mutated in DC (DKC1, NHP2, NOP10, and WRAP53/TCAB1) encode for proteins associated with abundant regulatory noncoding RNAs (ncRNAs) termed H/ACA small RNAs and form H/ACA small ribonucleoprotein (RNP) complexes involved in modifying ribosomal RNA (rRNA) and small nuclear RNA (snRNA) (30-32). H/ACA small RNAs are associated several important RNA-based cellular processes implicated in telomere, ribosome, and splicing biology (30,33). The pseudouridine synthase dyskerin, encoded by DKC1 gene, have effect on modification of pseudouridine residues on rRNA (30,34,35). The largest subgroup of dyskerin-associated H/ACA small RNAs, termed H/ACA small nucleolar RNAs (snoRNAs), is responsible for guiding dyskerin to convert uridine to pseudouridine at select nucleotides within the rRNA (36). Distinct DKC1 mutations lead to a significant decrease in the levels and activity of several H/ACA snoRNAs known to guide pseudouridine modifications on rRNA, and causing site-specific defects in rRNA pseudouridine modifications at distinct residues (37). In addition, pseudouridine modifications may play an important role in maintaining translation fidelity and modulating the expression of specific mRNAs harbor IRES elements such as p53 and p27 (38).
Current diagnosis and management of IBMFs in China
Understanding IBMFS
Because FA is the most common of the IBMFS, it should be excluded first before establishing a diagnosis of ABMFs. The Fanconi Anemia Research Foundation suggested indications for defining and testing for FA in 2008 (39). In clinical practice, it is critical to differentially diagnose FA from non-FA BMFs, chromosome fragility syndrome, hereditary diseases with congenital malformations and mental retardation and non-FA tumors. In fact, it is not difficult to diagnose FA in 70% of patients with congenital malformations, but in those without these malformations, physicians have a good chance of overlooking the subtle traits of the FA phenotype and misdiagnosing those patients with ABMFS, thus delaying prompt treatment (40).
Multicenter studies have shown that the five most common subsets of IBMFs are FA, DC, SDS, DBA and SCN; TAR and CAMT are relatively rare (16). Based on the survey records of 3956 patients with BMFS under 14 years of age who were enrolled in our center from June 2003 to October 2014, 293 (7%) patients were diagnosed with IBMFS, including 132 cases of DBA, 85 of FA, 57 of SCN, 12 of DC and 4 of SDS (unpublished); however, these results were not entirely consistent with those from other centers. A significant percentage of patients with IBMFS with single cytopenia show no congenital malformations, and physicians who are not fully aware of the complete spectrum of IBMFS might overlook these conditions in certain patients. For example, with DC, the typical mucocutaneous triad of abnormal skin pigmentation, nail dystrophy and mucosal leukoplakia might not be present before the onset of hematological abnormalities. In many instances, it is not uncommon for physicians to misdiagnose these patients with immune thrombocytopenia (ITP) or aplastic anemia and thus administer strengthened immunosuppressive therapy (IST). Moreover, to date, the number of reported cases may be less than the actual morbidity in China because of limitations in the detection approaches.
The clinical detection of IBMFs
Regular laboratory examinations include peripheral blood tests and bone marrow tests, but the test results are assumed to be different during the progression of the diverse diseases. With FA, the routine peripheral blood tests may indicate single cytopenia, bi-cytopenia, or pancytopenia, and the anemia should be macrocytic with elevated fetal hemoglobin (non-hemoglobinopathy). Yet, in the middle or late stages of this disease, bone marrow smears display notable hypoplastic features that affect megakaryocytes in particular. In cases of malignant transformation, specific clonal cytogenetic changes are detectable; for example, duplication of chromosomes 1q or3q and loss of the entire chromosome 7 indicate poor prognosis. In situ hybridization also reinforces the accuracy of clonal cytogenetic examinations. All of the above mentioned routine test for blood disease are capable of be running by the specialized and general hospitals, however, not available in many local hospitals in China. This may cause misdiagnosis and missed diagnosis occasionally in local hospitals. The golden standard test for FA is the chromosome breakage test (most common are the mitomycin C (MMC)-induced chromosomal fragile experiment and the diepoxybutane-induced chromosomal fragile experiment), which is often completed by various testing companies in China. Currently, the lack of standardized testing for rare IBMFS needs to be addressed and increasing the knowledge of non-hematologic physicians will greatly decrease the number of misdiagnosis.
Genetic and molecular diagnosis of IBMFs
Studies have discovered 16 genes with evidence for the existence of 16 complementary subtypes, and notably, there are relevant genes for the diagnosis of other rare IBMFS disorders (DC, SDS, DBA, SCN, TAR and CAMT). However, it is time-consuming and very expensive to establish the molecular and genetic technology related to amplify, sequencing and analyzing DNA samples. Most clinical diagnostic centers in China send their FA patient samples for genetic testing only when the chromosome breakage test is positive, similar to hematologists worldwide; otherwise, detecting the most common subtypes of FA (the FANCA, FANCC, and FANCG complementary subtypes are most common) is another cost-saving method. It is encouraging that the combined application of targeted capture and next-generation sequencing (NGS) has accelerated the accuracy and efficiency of molecular genetic examinations because the technology has dramatically reduced the required number of living cells and simplified the experimental procedures. Our center has discovered concomitant mutations in multiple FA genes in individual FA patients by performing full exome sequencing of peripheral blood specimens from FA patients and their family members, indicating that Chinese FA patients and carriers might have higher and more complex mutation rates in the FANC genes than has been conventionally recognized (41). It is conceivable that NGS will play a considerable role in elucidating the pathogenesis of diseases that we do not yet know about. Nevertheless, in clinical practice, we have found certain unexpected mismatches between a clinical condition and mutant genes in a single individual, which are difficult to explain. Currently, it remains unclear whether these discrepancies are due to the divergence and complexity of the human body or limited understanding. In the future, it will be challenging to manage vast amounts of bioinformatics data and complete the relevant functional verifications.
The clinical management of IBMFs
The clinical manifestations of IBMFs are diverse, and thus, the therapeutic effects of medication differ. It has been reported that 80% of DBA patients are sensitive to glucocorticoids at a starting dose of 2 mg/kg, but the therapy must be sustained for most patients. In our cohort of 50 pediatric patients with DBA, who were administrated with glucocorticoid (1 mg/kg), after median follow-up of 29 months, totally 32 (64%) patients had response. However, only 15 (30%) patients achieved complete remission without sustained therapy (42). Additionally, substantial side effects in skeletal development must be closely monitored in patients under one year of age; patients with DC or FA appear to be sensitive to androgens, but hematopoietic stem cell transplantation (HSCT) is the only definitive treatment for marrow failure. Europe (Italy and France), the United States and Asia (Korea) have established registries for DBA, FA, and other IBMFS and have prepared diagnostic and treatment guidelines for the benefit of incidence analyses, precise diagnoses, and short-and long-term follow-up treatment efficacy analyses as well as complication management (43,44). In China, we have made great progress in diagnostic precision and managing IBMFS, also providing informative materials and instructions for patients and their families. It has been suggested that comprehensive evaluations be performed at the time of diagnosis and that ongoing monitoring be tailored to each IBMFS patient to continuously improve their outcomes. Thus, there remains a long way for us to go to achieve the aim of precision medicine.
Improving the comprehensive diagnosis and management of IBMFS in China
Recently, there are a lot of clinical trials on the clinical features, prognosis and treatment for IBMFs. The clinical trial NCT00027274 is comparing differences between patients with IBMFS who develop cancer and those who don’t to determine risk of cancer in IBMFS patients with specific gene mutations. The clinical trial NCT01001598, NCT01034592 is identifying the safety and efficacy of danazol in patients with FA and DC, and lenalidomide in DBA, respectively. Previous study showed administrated growth hormone could improve short stature in children with DBA (45). And lots of trails is identifying the safety and efficacy of different sources of hematopoietic stem cell and conditioning regimen for HSCT for inherited bone marrow failure syndromes (IBMFs).
IBMFs are a set of relatively rare disorders, with clinical manifestations that include multiple physical deformities, tumor susceptibility and progressive bone marrow failure, and the management of IBMFs involves hematology, genetics, oncology and many other fields. The complexity of these disorders has posed great challenges for the accurate diagnosis and appropriate treatment of IBMFs for decades. The Fanconi Anemia International Registry (FAIR), which was founded in 1982, has played a major role in identifying the genotype and revealing the clinical features of FA. Since the first IBMFs registry department was established in the 1980s, a Dyskeratosis Congenita Registry was established at the Hammersmith Hospital of Imperial College of London in 1995, a DC-specific workshop was held at the NIH on September 19, 2008, and a DBA registry was subsequently established (46-48). All the scientific and medical fields have made significant contributions to research on the pathogenesis, diagnosis and treatment of IBMFs. Recently, enormous efforts have been made to establish our own IBMFs database in China because there are insufficient precise data on these diseases. Meanwhile, it will be useful for the government to organize epidemiological investigations and enforce prenatal screening on a national scale with the aim of reducing birth defects, best utilizing social resources and improving population health.
Furthermore, the actual incidence of IBMFs in China may be much higher than that obtained from our clinically diagnosed cases because precise data on the national incidence rates remain unavailable. Finally, we should not only establish a pathological cell bank to improve diagnostics, investigate pathogenesis and discover targeted therapies but also closely integrate fundamental research and clinical practice to improve the comprehensive diagnosis and management of IBMFs in China.
Acknowledgements
Funding: This work was supported by grants from the National Natural Science Foundation of China [81170470] and from the key project for Ministry of Health hospitals [2010-2012].
Footnote
Conflicts of Interest: The author has no conflicts of interest to declare.
References
- Biswajit H, Pratim PP, Kumar ST, et al. Aplastic anemia: a common hematological abnormality among peripheral pancytopenia. N Am J Med Sci 2012;4:384-8. [PubMed]
- Young NS, Calado RT, Scheinberg P. Current concepts in the pathophysiology and treatment of aplastic anemia. Blood 2006;108:2509-19. [PubMed]
- Găman A, Găman G, Bold A. Acquired aplastic anemia: correlation between etiology, pathophysiology, bone marrow histology and prognosis factors. Rom J Morphol Embryol 2009;50:669-74. [PubMed]
- Subspecialty Group of Hematology; Society of Pediatrics; Chinese Medical Association The Editorial Board; Chinese Journal of Pediatrics. Recommendations for diagnosis and treatment of acquired aplastic anemia in children. Zhonghua Er Ke Za Zhi 2014;52:103-6. [PubMed]
- Auerbach AD. Fanconi anemia and its diagnosis. Mutat Res 2009;668:4-10. [PubMed]
- Touzot F, Le Guen T, de Villartay JP, et al. Dyskeratosis congenita: short telomeres are not the rule. Med Sci (Paris) 2012;28:618-24. [PubMed]
- Kathuria R, Poddar U, Yachha SK. Shwachman-Diamond Syndrome: are we missing many? Indian Pediatr 2012;49:748-9. [PubMed]
- Geddis AE. Congenital amegakaryocytic thrombocytopenia. Pediatr Blood Cancer. 2011;57:199-203. [PubMed]
- Teo JT, Klaassen R, Fernandez CV, et al. Clinical and genetic analysis of unclassifiable inherited bone marrow failure syndromes. Pediatrics 2008;122:e139-48. [PubMed]
- Schifferli A, Kühne T. Fanconi Anemia: Overview of the Disease and the Role of Hematopoietic Transplantation. J Pediatr Hematol Oncol 2015;37:335-43. [PubMed]
- Kirwan M, Dokal I. Dyskeratosis congenita: a genetic disorder of many faces. Clin Genet 2008;73:103-12. [PubMed]
- Vlachos A, Ball S, Dahl N, et al. Diagnosing and treating Diamond Blackfan anaemia: results of an international clinical consensus conference. Br J Haematol 2008;142:859-76. [PubMed]
- Myers KC, Bolyard AA, Otto B, et al. Variable clinical presentation of Shwachman-Diamond syndrome: update from the North American Shwachman-Diamond Syndrome Registry. J Pediatr 2014;164:866-70. [PubMed]
- Giri N, Alter BP, Penrose K, et al. Immune status of patients with inherited bone marrow failure syndromes. Am J Hematol 2015;90:702-8. [PubMed]
- Parikh S, Bessler M. Recent insights into inherited bone marrow failure syndromes. Curr Opin Pediatr 2012;24:23-32. [PubMed]
- Alter BP. Inherited bone marrow failure syndromes. In: Nathan DG, Ginsburg D, Orkin SH, Look AT, editors. Nathan and Oski’s Hematology of Infancy and Childhood. 6th ed. Philadelphia: Saunders; 2003:280-365.
- Zhang JY, An WB, Zhang L, et al. Genotype analysis and telomere length measure in patients with dyskeratosis congenita. Zhongguo Shi Yan Xue Ye Xue Za Zhi 2015;23:212-6. [PubMed]
- Gao R, Chen S, Kobayashi M, et al. Bmi1 promotes erythroid development through regulating ribosome biogenesis. Stem Cells 2015;33:925-38. [PubMed]
- Léger-Silvestre I, Caffrey JM, Dawaliby R, et al. Specific Role for Yeast Homologs of the Diamond Blackfan Anemia-associated Rps19 Protein in Ribosome Synthesis. J Biol Chem 2005;280:38177-85. [PubMed]
- Choesmel V, Bacqueville D, Rouquette J, et al. Impaired ribosome biogenesis in Diamond-Blackfan anemia. Blood 2007;109:1275-83. [PubMed]
- Flygare J, Aspesi A, Bailey JC, et al. Human RPS19, the gene mutated in Diamond-Blackfan anemia, encodes a ribosomal protein required for the maturation of 40S ribosomal subunits. Blood 2007;109:980-6. [PubMed]
- Robledo S, Idol RA, Crimmins DL, et al. The role of human ribosomal proteins in the maturation of rRNA and ribosome production. RNA 2008;14:1918-29. [PubMed]
- Donati G, Peddigari S, Mercer CA, et al. 5S ribosomal RNA is an essential component of a nascent ribosomal precursor complex that regulates the Hdm2-p53 checkpoint. Cell Rep 2013;4:87-98. [PubMed]
- Payne EM, Virgilio M, Narla A, et al. L-Leucine improves the anemia and developmental defects associated with Diamond-Blackfan anemia and del(5q) MDS by activating the mTOR pathway. Blood 2012;120:2214-24. [PubMed]
- Han JM, Jeong SJ, Park MC, et al. Leucyl-tRNA synthetase is an intracellular leucine sensor for the mTORC1-signaling pathway. Cell 2012;149:410-24. [PubMed]
- Boultwood J, Yip BH, Vuppusetty C, et al. Activation of the mTOR pathway by the amino acid (L)-leucine in the 5q- syndrome and other ribosomopathies. Adv Biol Regul 2013;53:8-17. [PubMed]
- Menne TF, Goyenechea B, Sánchez-Puig N, et al. The Shwachman-Bodian-Diamond syndrome protein mediates translational activation of ribosomes in yeast. Nat Genet 2007;39:486-95. [PubMed]
- Finch AJ, Hilcenko C, Basse N, et al. Uncoupling of GTP hydrolysis from eIF6 release on the ribosome causes Shwachman-Diamond syndrome. Genes Dev 2011;25:917-29. [PubMed]
- Wong CC, Traynor D, Basse N, et al. Defective ribosome assembly in Shwachman-Diamond syndrome. Blood 2011;118:4305-12. [PubMed]
- Kiss T, Fayet-Lebaron E, Jády BE. Box H/ACA small ribonucleoproteins. Mol Cell 2010;37:597-606. [PubMed]
- Matera AG, Terns RM, Terns MP. Non-coding RNAs: lessons from the small nuclear and small nucleolar RNAs. Nat Rev Mol Cell Biol 2007;8:209-20. [PubMed]
- Terns M, Terns R. Noncoding RNAs of the H/ACA family. Cold Spring Harb Symp Quant Biol 2006;71:395-405. [PubMed]
- Meier UT. The many facets of H/ACA ribonucleoproteins. Chromosoma 2005;114:1-14. [PubMed]
- Ruggero D, Grisendi S, Piazza F, et al. Dyskeratosis congenita and cancer in mice deficient in ribosomal RNA modification. Science 2003;299:259-62. [PubMed]
- Gu BW, Bessler M, Mason PJ. A pathogenic dyskerin mutation impairs proliferation and activates a DNA damage response independent of telomere length in mice. Proc Natl Acad Sci U S A 2008;105:10173-8. [PubMed]
- Ni J, Tien AL, Fournier MJ. Small nucleolar RNAs direct site-specific synthesis of pseudouridine in ribosomal RNA. Cell 1997;89:565-73. [PubMed]
- Bellodi C, McMahon M, Contreras A, et al. H/ACA small RNA dysfunctions in disease reveal key roles for noncoding RNA modifications in hematopoietic stem cell differentiation. Cell Rep 2013;3:1493-502. [PubMed]
- Jack K, Bellodi C, Landry DM, et al. rRNA pseudouridylation defects affect ribosomal ligand binding and translational fidelity from yeast to human cells. Mol Cell 2011;44:660-6. [PubMed]
- Eiler ME, Frohnmayer D, Frohnmayer L, et al. Fanconi Anemia: Guidelines for Diagnosis and Management. 3 ed. Eugene, OR: Fanconi Anemia Research Fund, Inc, 2008.
- Giampietro PF, Verlander PC, Davis JG, et al. Diagnosis of Fanconi anemia in patients without congenital malformations: an international Fanconi Anemia Registry Study. Am J Med Genet 1997;68:58-61. [PubMed]
- Chang L, Yuan W, Zeng H, et al. Whole exome sequencing reveals concomitant mutations of multiple FA genes in individual Fanconi anemia patients. BMC Med Genomics 2014;7:24. [PubMed]
- Chen Y, Liu T, Ruan M, et al. Clinical Analysis of Diamond-Blackfan Anemia. International Journal of Blood Transfusion and Hematology. 2014;37:406-11.
- Vlachos A, Klein GW, Lipton JM. The Diamond Blackfan Anemia Registry: tool for investigating the epidemiology and biology of Diamond-Blackfan anemia. J Pediatr Hematol Oncol 2001;23:377-82. [PubMed]
- Auerbach AD, Schroeder TM. First announcement of the Fanconi anemia International Registry. Blood 1982;60:1054. [PubMed]
- Howell JC, Joshi SA, Hornung L, et al. Growth hormone improves short stature in children with Diamond-Blackfan anemia. Pediatr Blood Cancer 2015;62:402-8. [PubMed]
- Lipton JM, Atsidaftos E, Zyskind I, et al. Improving clinical care and elucidating the pathophysiology of Diamond Blackfan anemia: an update from the Diamond Blackfan Anemia Registry. Pediatr Blood Cancer 2006;46:558-64. [PubMed]
- Savage SA, Dokal I, Armanios M, et al. Dyskeratosis congenita: the first NIH clinical research workshop. Pediatr Blood Cancer 2009;53:520-3. [PubMed]
- Knight S, Vulliamy T, Copplestone A, et al. Dyskeratosis Congenita (DC) Registry: identification of new features of DC. Br J Haematol 1998;103:990-6. [PubMed]
Cite this article as: Zhu X. Current insights into the diagnosis and treatment of inherited bone marrow failure syndromes in China. Stem Cell Investig 2015;2:15.