Expression of inflammatory cytokines in mesenchymal stem cells derived from proximal humerus fractures
Introduction
The availability and versatility of the mesenchymal stem cells (MSCs) make them an excellent treatment option for a wide variety of orthopaedic conditions, by enhancing the healing process in osteoarthrosis, osteochondritis, chondromalacia, pseudarthrosis, bone losses, and nerve lesions in the spinal cord trauma (1).
The products secreted by MSCs, known as secretome, can be used for severe fracture treatment with consolidation difficulties (2). The immunomodulatory properties of MSCs can vary based on their environment. Many cytokines and growth factors secreted by MSCs confer not only considerable stability and flexibility to the cells but also a prompt amplification of the response to a particular stimulus. An unbalance in this network, caused either by progressive diseases, bacterial and viral infections, or even fractures, may modify the intrinsic biological functions of stem cells (3).
Traditionally, bone marrow (BM) MSCs are obtained from the iliac crest or proximal femur, without posing a risk to the donor site. However, obtaining MSCs from fractures, such as those affecting the humerus, can allow the collection and expansion of these cells without the need for another intervention in these subgroups of orthopaedic patients.
This study evaluates if BM harvested during surgical procedures on proximal humerus fractures can be useful to obtain MSCs to be applied in bone regenerative therapies, assessing the in vitro profile of the main inflammatory cytokines produced by these cells. We present the following article in accordance with the STROBE reporting checklist (available at https://sci.amegroups.com/article/view/10.21037/sci-2021-031/rc).
Methods
The study protocol was approved by the Institutional Research Ethics Committee (ERB number 1.376.415) and was conducted in accordance with the tenets of the Declaration of Helsinki (as revised in 2013). Informed consent was obtained from all participants.
Two healthy adult patients with proximal humerus fractures who underwent osteosynthesis treatment within 48 h of the trauma were included.
The donor patients were a 46-year-old female and a 65-year-old male. None of them had any complications during the BM harvesting procedure or in the post-operative fracture consolidation phase.
Exclusion criteria were open fractures, infection, or pathological fractures.
BM harvesting
Before osteosynthesis surgery, 15 mL of BM was collected from the humerus diaphysis, at least 5 cm from the fracture situs, using a Jamshid needle (14-gauge) connected to a syringe containing 1 mL (1,000 U) of sodium heparin. The syringe was gently stirred to mix the heparin with the BM. The samples were maintained on ice and immediately transferred to the laboratory for pre-processing the BM.
Establishment of MSC primary cultures
The BM aspiration was diluted in three volumes of Dulbecco’s Modified Eagle’s Medium/Nutrient mixture F-12 Ham (DMEM/F12; Gibco, Grand Island, NY, USA). Six mL of diluted BM was layered over 3 mL of Ficoll-Paque Plus (GE Healthcare Life Science, Freiburg, Germany) and centrifuged at 1,600 rpm for 30 min. The white cloudy interface between the Ficoll and the culture medium was collected, pooled into a 15-mL tube, suspended in DMEM/F12 nutrient medium, and then washed twice. Cells were suspended in DMEM/F12 and seeded in two 25 cm2 culture flasks, then incubated at 37 °C and 5% CO2 with mesenchymal stem cell basal medium (MSCBM-CD Lonza, Walkersville, MD, USA) supplemented with 20% of foetal calf serum (FCS) (Gibco, Grand Island, NY, USA). After 48 h, non-adherent cells were washed away. Nutrient medium was changed every 4 days until cells reached 80–90% confluence, at which point they were detached with 0.25% trypsin solution (Gibco, Grand Island, NY, USA) and seeded at a density of 500 cells/cm2 in 75 cm2 culture flasks until the third passage (4). Cells were characterized as MSCs by phenotypic and functional criteria, observing their ability to adhere to the plastic surface, to express specific cell surface antigens and the potential to differentiate into lineages of mesenchymal tissues, including osteocytes and adipocytes (5).
MSC phenotyping
Third passage MSCs were phenotypically characterized by flow cytometry using a panel of markers including CD73, CD90, CD105, CD34, CD45, CD11b, CD19, and human leukocyte antigen (HLA)-DR directly conjugated to fluorophores (BD Stemflow hMSC Analysis Kit, Becton Dickinson Pharmingen, Sparks, MD, USA). Briefly, cells were detached using 0.25% trypsin solution and the cell suspension was adjusted to [5–10] ×106 cells/mL. Cells were dispensed into microtubes and mixed with monoclonal antibodies or their respective isotypic controls (Table S1). Next, 100 µL of the cell suspension was dispensed into each microtube, mixed with the appropriate monoclonal antibody or respective isotypic control, and incubated for 30 min at 2–8 °C, protected from light. Cells were washed twice with cold PBS containing 10% FCS, resuspended in 250 µL of this same solution, and placed in cytometry tubes. The data were immediately acquired with a FACS Canto II (BD Biosciences, San Jose, CA, EUA) flow cytometer and analysed using the Flow Jo software (Tree Star, Ashland, OR, USA).
In vitro differentiation of MSCs into osteocytes and adipocytes
For osteogenic differentiation, 3×103 cells/cm2 of the third passage MSCs were plated on a 10-mm round coverslip, in 24-well plates, with MSCBM nutrient medium (Lonza, Walkersville, MD, USA). Cells were cultured at 37 °C with 5% CO2 for 24 h to adhere before undergoing nutrient exchange. Four control wells were filled only with MSCBM, whereas the other four remaining wells were induced to form mineral deposits with human MSC (hMSC) Osteogenic Differentiation Medium (Lonza, Walkersville, MD, USA), containing DMEM culture medium supplemented with dexamethasone, glycerol phosphate, ascorbic acid, sodium pyruvate, proline, L-glutamine, gentamicin, amphotericin and ITS (insulin, transferrin and selenium) every 3 days, for 24 days. Calcium deposition was identified by histochemical staining with Alizarin Red S (Sigma-Aldrich, St. Louis, MO, USA). The cell monolayer was fixed with 4% paraformaldehyde (PFA), washed with ultrapure water, and immersed in Alizarin Red S solution for 5 min. Excess dye was removed, and monolayers were washed three times with ultrapure water. Coverslips were removed from plates, placed on a microscopy coverslip, and analyzed by standard microscopy.
For adipogenic differentiation, the third passage MSCs at a density of 2×104 cells/cm2 were plated in a 24-well plate, on round coverslips as mentioned above. Cells were cultured in MSCGM nutrient medium for 7 days until reaching confluence. Control cultures were kept in MSCGM medium, while adipogenesis was induced by culturing in hMSC Adipogenic Differentiation Medium (Lonza, Walkersville, MD, USA), containing DMEM culture medium supplemented with h-insulin, L-glutamine, mesenchymal cell growth supplements (MCGS), dexamethasone, indomethacin, 3-isobutyl-1-methyl-xanthine (EBMX), and gentamicin. The Adipogenic Differentiation Medium was alternated with the hMSC Adipogenic Maintenance Medium (Lonza, Walkersville, MD, USA) containing MCGS medium with h-insulin, L-glutamine, gentamicin, and penicillin every 3 days for 13 days. Adipogenesis was confirmed by histochemical staining of fat vacuoles with Oil Red O. Briefly, cells were fixed with 4% PFA for 30 min, washed with PBS and incubated with Oil Red O solution (Sigma-Aldrich, St. Louis, MO, USA) for 2 h. Coverslips with the cells were removed, washed three times with ultrapure water, rested on a microscopy coverslip and analyzed by standard microscopy.
Inflammatory cytokine secretion in MSC derived from humerus fractures
The production of inflammatory cytokines was assessed by enzyme-linked immunosorbent assays (ELISA), from the conditioned medium of 3.8×104 third passage MSCs cultured for 3, 5 and 7 days.
Conditioned culture media were collected and tested using commercial ELISA kits designed to quantify interleukin (IL)-1β, IL-6, IL-8, IL-10, tumor necrosis factor α (TNF-α), and interferon γ (IFN-γ) (R&D Systems, Minneapolis, MN, USA), according to the manufacturer’s instructions. Samples in which cytokine levels were estimated to be below the sensitivity of the assay were set as equal to the sensitivity of the assay and those with concentrations above the standard curve were diluted and re-assayed. Assay outcomes were recorded on an automatic reader (Epoch-BioTek, Winooski, VT, USA) at a wavelength of 492 nm. The concentration of the respective cytokines in the conditioned culture medium was calculated according to a standard curve. To obtain measurable readings for IL-8, the conditioned medium was diluted to 1:10.
Statistical analysis
Data were generated from primary culture experiments with triplicates. Graphical and statistical analyses were performed using Graph Pad Prism 9.0 (Graph Pad Software, San Diego, CA, USA). Grouped data are reported as mean ± standard error of mean (SEM). Significance was evaluated by Kruskal-Wallis test and accepted when P values were ≤0.05.
Results
MSC primary cultures
After 3 days in culture, adherent cells with spindle-shaped morphology were observed. MSCs proliferated and reached confluence in 10 days, when the first cell passage was performed. The second cell passage was performed after 5 days (Figure 1A).
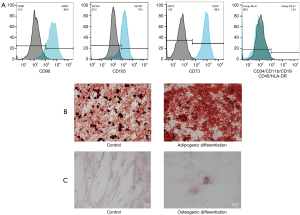
MSC phenotyping
Phenotypic characterization by flow cytometry demonstrated that cultured cells were consistent with populations of MSCs, characterized by cell size and granularity. Analysed cells (Figure 1B) showed high expression of the stem cell markers CD90 (average of 83.5%), CD105 (average of 75.4%) and CD73 (average of 96.1%) and negative or very low expression of CD34, CD45, CD11b, CD19, and HLA-DR (average of 1.54%), which were used to identify potential contaminants, such as hematopoietic stem cells. These results validated our approach to obtain a homogeneous culture of human BM MSCs.
In vitro differentiation of MSCs into osteocytes and adipocytes
MSCs were induced to differentiate into adipocytes stained with Oil Red O and showed numerous lipidic vesicles in the cytoplasm. The control cells showed only the lipid of plasma membranes (Figure 1C). MSCs induced to differentiate into osteocytes were stained with Alizarin Red S, which showed mineralized nodules of bone matrix and calcium deposits. The non-induced control cells maintained the elongated MSC morphology (Figure 1C).
Inflammatory cytokine evaluation
The profile of the secreted cytokine in the conditioned culture medium is shown in Figure 2. The comparison of expression levels demonstrated that IL-8 was the most secreted cytokine, followed by IL-6 and IL-10 at similar quantities. The IL-1β was the lower secreted cytokine, while TNF-α and IFN-γ were not detected (Figure 2A). There was no statistically significant difference in cytokine production after 3, 5, or 7 days (P>0.05), as detailed in Figure 2B. However, there was a trend toward increased levels of IL-8 secretion on day 7 (P=0.0667).
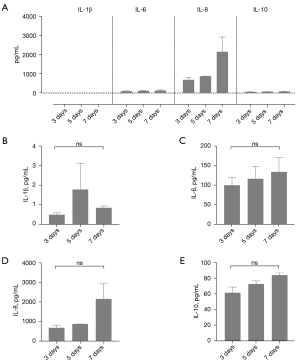
Discussion
According to our results, it is possible to successfully obtain MSC cultures from BM collected during osteosynthesis surgeries on fractured proximal humeri. As these cells rapidly proliferate, the cells or their secreted products can be used in patients with consolidation problems or can even be used to maintain a stem cell bank.
Although BM harvested from the iliac crest is still considered the gold standard (6), the use of other sites for MSC collection can have advantages. The MSCs obtained from BM aspiration during arthroscopic surgery to repair the proximal humerus or distal femur fracture certainly reduce the need for further surgical procedures (7), avoiding risks of increased pain, infection, postoperative bleeding, and neurovascular damage if the donor situs is the iliac crest (8).
Our results showed high expression of CD73 (96.1%), CD105 (75.4%), and CD90 (83.5%) and negative or very low expression of CD34, CD45, CD11b, CD19, and HLA-DR (average of 1.54%). The hMSC population measured by flow cytometry must be highly positive for the surface markers CD73, CD90 and CD105. Additionally, the markers CD34, CD45, and CD14 must be expressed in less of 2% of the cell population (9).
Differentiation assays with Oil Red O showed that cells were able to differentiate into adipocytes, accumulating a large number of lipid vesicles in the cytoplasm. Alizarin Red S staining showed calcium nodules of bone matrix in the cells that were induced to differentiate into osteoblasts. This ability to differentiate into adipocytes and osteoblasts, combined with the immunophenotyping results, characterize the cultured cells as human BM MSCs.
We studied the main inflammatory cytokines produced by the MSC cultures harvested from the fractured proximal humerus of patients to determine whether the cytokine secretion by cells collected from those sites can have similar kinetics from those collected from healthy iliac crest.
Our study showed that IL-8 was the most-produced cytokine, followed by the IL-6 cytokine. Although the baseline levels of these cytokines in healthy controls are largely unknown (10), the cytokine secretion profile in human non-stimulated BM MSC cell lines is IL-6 followed by IL-8 (4).
The IL-6 is responsible for the maintenance of BM MSCs in their undifferentiated state (11), acting as anti-inflammatory and regenerative response mediator and plays a major role in fracture healing (12). The altered IL-6 produced by our MSC probably resulted from the previous bone injury stimulus. Therefore, a different cytokine profile from the healthy iliac crest was seen, with the major secretion of IL-8, surpassing the IL-6 production. However, IL-8 has chemo-attractant effects on bone progenitor cells, being very beneficial for bone repair. Fracture human hematomas, which contain all the required signals to initiate and actively promote the formation of a new bone also has IL-8 as one of the most secreted cytokines, instead of IL-6 production (10).
We observed lower levels of IL-10 and IL-1β cytokines produced by the MSCs, the same profile of cytokines of fracture haematomas acute phase, and contrasting with the production profile of BM MSCs harvested from iliac crests, which do not produce IL-10, even after inflammatory stimulation with IL-1β, IL-6, and IL-23 (13). IL-10 is a potent anti-inflammatory cytokine that regulates the intensity of inflammation and exerts a direct effect on osteogenesis (14). Conversely, IL-1β is a pro-inflammatory acute phase cytokine that induces the secretion of other cytokines, such as IL-6, and it has been shown that IL-1β can enhance the proliferation of human osteoblasts in vitro (15).
The MSCs in the current study were harvested within 48 h of the injury. The acute inflammatory response after injury peaks within the first 24–48 h and is generally complete after 7 days (16). As the cytokine profile secreted by these cells is different from that of non-stimulated BM MSCs and very similar to that of acute phase hematomas (which have the essential activation factors for initiating bone regeneration), our results showed that the culture and expansion of MSCs harvested from proximal humerus fractures are a possible alternative and this may also have the advantage of allowing the culture of already-activated MSCs from the injury site, thus optimizing the cells for use when a prompt bone regeneration effect is necessary.
The limitation of this study is the small sample size, but our main purpose was to describe the profile of the secreted cytokines involved in MSCs inflammatory processes and not to evaluate the donor’s variables implicated in its expression levels.
Further studies on a wider range of inflammatory cytokines can provide a better understanding of the real benefits of harvesting MSCs from fracture situs, since we were limited only to the main cytokines commonly known to be involved in post-injury inflammatory processes.
Conclusions
Our results evidence that the humerus proximal fracture situs is a potential alternative site for collecting MSCs, being a safe and reproducible technique. The main cytokines secreted by the cultured MSCs from the fracture situs were IL-8, IL-6, IL-10, and IL-1β. These findings indicate that MSCs derived from humerus fractures has the potential to enhance the healing process of bone fractures.
Acknowledgments
Funding: This work was supported by the Fundação de Amparo a Pesquisa do Estado de Sao Paulo (FAPESP) (grant number 2015/10727-0 to SAS), and by Universidade Estadual Paulista (UNESP) Research Rectory (grant number 169 to MMHV).
Footnote
Reporting Checklist: The authors have completed the STROBE reporting checklist. Available at https://sci.amegroups.com/article/view/10.21037/sci-2021-031/rc
Conflicts of Interest: All authors have completed the ICMJE uniform disclosure form (available at https://sci.amegroups.com/article/view/10.21037/sci-2021-031/coif). The authors have no conflicts of interest to declare.
Ethical Statement: The authors are accountable for all aspects of the work in ensuring that questions related to the accuracy or integrity of any part of the work are appropriately investigated and resolved. This study was conducted in accordance with the Declaration of Helsinki (as revised in 2013) and was approved by the Research Ethics Committee, Botucatu Medical School, UNESP (ERB number 1.376.415) and informed consent was taken from all voluntary patients.
Open Access Statement: This is an Open Access article distributed in accordance with the Creative Commons Attribution-NonCommercial-NoDerivs 4.0 International License (CC BY-NC-ND 4.0), which permits the non-commercial replication and distribution of the article with the strict proviso that no changes or edits are made and the original work is properly cited (including links to both the formal publication through the relevant DOI and the license). See: https://creativecommons.org/licenses/by-nc-nd/4.0/.
References
- Berebichez-Fridman R, Gómez-García R, Granados-Montiel J, et al. The Holy Grail of Orthopedic Surgery: Mesenchymal Stem Cells-Their Current Uses and Potential Applications. Stem Cells Int 2017;2017:2638305. [Crossref] [PubMed]
- Teixeira FG, Salgado AJ. Mesenchymal stem cells secretome: current trends and future challenges. Neural Regen Res 2020;15:75-7. [Crossref] [PubMed]
- Park CW, Kim KS, Bae S, et al. Cytokine secretion profiling of human mesenchymal stem cells by antibody array. Int J Stem Cells 2009;2:59-68. [Crossref] [PubMed]
- Ramakrishnan A, Torok-Storb B, Pillai MM. Primary marrow-derived stromal cells: isolation and manipulation. Methods Mol Biol 2013;1035:75-101. [Crossref] [PubMed]
- Pittenger MF, Mackay AM, Beck SC, et al. Multilineage potential of adult human mesenchymal stem cells. Science 1999;284:143-7. [Crossref] [PubMed]
- Vasiliadis AV, Galanis N. Human bone marrow-derived mesenchymal stem cells from different bone sources: a panorama. Stem Cell Investig 2020;7:15. [Crossref] [PubMed]
- Beitzel K, McCarthy MB, Cote MP, et al. Comparison of mesenchymal stem cells (osteoprogenitors) harvested from proximal humerus and distal femur during arthroscopic surgery. Arthroscopy 2013;29:301-8. [Crossref] [PubMed]
- Banwart JC, Asher MA, Hassanein RS. Iliac crest bone graft harvest donor site morbidity. A statistical evaluation. Spine (Phila Pa 1976) 1995;20:1055-60. [Crossref] [PubMed]
- Dominici M, Le Blanc K, Mueller I, et al. Minimal criteria for defining multipotent mesenchymal stromal cells. The International Society for Cellular Therapy position statement. Cytotherapy 2006;8:315-7. [Crossref] [PubMed]
- Pountos I, Walters G, Panteli M, et al. Inflammatory Profile and Osteogenic Potential of Fracture Haematoma in Humans. J Clin Med 2019;9:47. [Crossref] [PubMed]
- Pricola KL, Kuhn NZ, Haleem-Smith H, et al. Interleukin-6 maintains bone marrow-derived mesenchymal stem cell stemness by an ERK1/2-dependent mechanism. J Cell Biochem 2009;108:577-88. [Crossref] [PubMed]
- Prystaz K, Kaiser K, Kovtun A, et al. Distinct Effects of IL-6 Classic and Trans-Signaling in Bone Fracture Healing. Am J Pathol 2018;188:474-90. [Crossref] [PubMed]
- Pourgholaminejad A, Aghdami N, Baharvand H, et al. The effect of pro-inflammatory cytokines on immunophenotype, differentiation capacity and immunomodulatory functions of human mesenchymal stem cells. Cytokine 2016;85:51-60. [Crossref] [PubMed]
- Walters G, Pountos I, Giannoudis PV. The cytokines and micro-environment of fracture haematoma: Current evidence. J Tissue Eng Regen Med 2018;12:e1662-77. [Crossref] [PubMed]
- Cho TJ, Gerstenfeld LC, Barnes GL, et al. Cytokines and fracture healing. Cur Opin Orthop 2001;12:403-8. [Crossref]
- Maruyama M, Rhee C, Utsunomiya T, et al. Modulation of the Inflammatory Response and Bone Healing. Front Endocrinol (Lausanne) 2020;11:386. [Crossref] [PubMed]
Cite this article as: Viveiros MMH, Viveiros MEDM, Silva MG, Kaneno R, Avelino NP, Rainho CA, Schellini SA. Expression of inflammatory cytokines in mesenchymal stem cells derived from proximal humerus fractures. Stem Cell Investig 2022;9:3.