Inflammation-induced emergency megakaryopoiesis: inflammation paves the way for platelets
Classical model of hematopoietic system has assumed that self-renewing hematopoietic stem cells (HSCs) exist at the apex of developmental hierarchy and give rise to all of highly specialized hematopoietic cells. In this model, lineage commitment is accompanied by decreasing self-renewing capacity; self-renewing HSCs transit into transiently amplifying multipotent progenitors with full lymphomyeloid potentials, followed by lineage specification. Recent researches, however, have cast doubt on this simplified model and there still exists considerable uncertainty regarding hematopoietic development.
First, when does lineage specification into megakaryocyte occur? It had been widely presumed that the first lineage specification occurs at a bifurcation between myeloid (CMPs: common myeloid progenitors) and lymphoid lineages (CLPs: common lymphoid progenitors) (1,2) and CMPs with restricted myeloid potential undergo next bifurcation into granulocyte-macrophage progenitors (GMPs) and megakaryocyte-erythroid (MegE) progenitors (MEPs). MEPs subsequently give rise to unipotent megakaryocyte progenitors (MkPs) as well as erythroid progenitors. But the study by Adolfsson et al. has generated controversy when they showed that Flt3high CD34+ fraction of Lineage− Sca-1+ c-kit+ cells (LSKs), called lymphoid-primed multipotent progenitors (LMPPs), sustain granulocyte, monocyte, B and T cell potentials, but lack significant MegE potential, predominantly by in vitro assays (3). The proposition that HSCs may directly give rise to MegE progenitors without passing through CMPs, raised by this seminal work, has been challenged by subsequent studies. Among them, in vivo approaches to assay MegE potential using hematopoietic cells from β-actin green fluorescent protein (GFP)-transgenic mice demonstrated LMPPs retained megakaryocyte potential, in spite of the fact that relatively higher doses were required for robust platelet production (4). In addition, studies using dual-color reporter mice model, in which all hematopoietic cells express red fluorescent protein and expression of Flt3 leads to GFP expression by Cre-mediated excision, revealed almost all blood lineages including platelets transit through an Flt3+ state (5). There have been still more significant studies in this field often accompanied by discrepant results, as extensively reviewed by a recent paper (6). Generally, results from these fate-mapping studies should be interpreted with caution for several reasons as below. Differentiation in vitro can be substantially affected by the culture conditions. Emergence of hematopoietic lineages in vivo transplantation experiments can be affected by the number of cells transplanted and the timing of the assay, especially when cells with low proliferative potential are assessed. Apparently single population can be heterogeneous, consisting of different subsets, generally with gradual transition. Only a very transient upregulation of Flt3 can lead to Cre-mediated excision. Furthermore, recent evidence has demonstrated considerable differences in cellular origins of hematopoietic cells are generated when they are reconstituted through transplantation, possibly reflecting tremendous stresses conferred by these assays (7). Despite these facts, several lines of evidence seem to wholly support the existence of MPP population with lymphoid and granulocyte-monocyte, but limited megakaryocyte-erythrocyte potential, which indicates a bypass of some other intermediate states from HSCs toward MegE progenitors. Along the same lines, a recent work has demonstrated the bypass directly by use of single-cell transplantation assay; Yamamoto et al. have identified myeloid-restricted progenitors with long-term self-renewing activity (MyRPs) in the phenotypic HSCs, which always possess megakaryocyte, with variable erythroid and myeloid potential (8), though the physiological relevance of the megakaryocyte-bias and the bypass pathway remains to be elucidated.
Secondly, though it has been long appreciated that megakaryocytes share a number of features with HSCs, such as responses to cytokines (9), cell surface receptors (10) and gene expression signatures (11), origins of the similarity between megakaryocytes and HSCs have been unclear. Recent progress in single cell analyses has elucidated that HSCs are functionally heterogeneous (12,13), and the most primitive HSCs at the apex of the hierarchy are platelet-biased, using von Willebrand factor (vWF)-eGFP reporter mice (14). In the study by Sanjuan-Pla et al. (14), vWF+ HSCs, megakaryocyte-primed HSCs with long-term myeloid lineage bias and only short-term lymphoid potential, were shown to self-renew and give rise to lymphoid-biased HSCs. Subsequent studies also revealed that a c-Kithigh subset of highly purified LT-HSCs (CD34− CD150+ LSK) was megakaryocyte-biased, though these studies demonstrated that c-Kithigh LT-HSCs were rather derived from c-Kitlow/int LT-HSCs (15). Taken together, though at least some part of HSCs are associated with intrinsic megakaryocyte-bias, relationships between each fraction reported to date, including above-mentioned MyRPs, remains unclear, as molecular characterization of these cells is lacking.
In addition to steady-state hematopoiesis mentioned above, another major challenge in hematopoietic stem cell biology is to clarify factors contributing to remarkable adaptability of hematopoietic system, especially HSCs, to various hematological stresses such as an inflammation, to ensure proper blood production. Among wide array of stress-driven hematopoiesis, myelopoietic response to acute systemic bacterial infection is a prime example, of which mechanisms have been extensively investigated. In this process, as covered in a recent review (16), hematopoietic stem and progenitor cells sense hematopoietic stresses via various mechanisms: depletion of downstream neutrophils, inflammatory cytokines, binding of pathogen-associated molecular patterns to toll-like receptors and paracrine signals from bone marrow niche cells. Though it was mainly appreciated that perception of these stimuli, mainly via cytokine production, leads to survival and proliferation of lineage committed progenitors, the last decade has witnessed an accumulating evidence of active involvement of uncommitted HSCs in response to inflammatory signals (17,18). As an example of mechanistic basis of response of HSCs, interferon-α-mediated cell cycle entry of quiescent HSCs is associated with post-transcriptional induction of c-Myc (19). Given that this kind of post-transcriptional regulation is assumed to be a rare event in steady-state HSCs, as indicated by a strong positive correlation between transcriptome and proteome data during lineage commitment (20), molecular insights into post-transcriptional regulation of gene expression in HSCs are largely unknown. In addition, although an association between inflammation and thrombocytosis is widely recognized and effects of inflammatory cytokines, including IFN-γ, on megakaryopoiesis have been investigated (21), not much is available on molecular mechanisms of inflammation-induced megakaryopoiesis.
Haas et al. addressed these issues in a recent article in Cell Stem Cell (22). Using high-throughput single-cell ex vivo lineage tracking, they identified a distinct subset within phenotypic HSCs, stem cell-like megakaryocyte-committed progenitors (SL-MkPs), which can generate only megakaryocyte colonies in vitro, in accordance with the work by Yamamoto et al. (8). This sophisticated system, in which single LT-HSCs were cultured in differentiation medium containing fluorescently labeled antibodies, enabled them to simultaneously analyze for morphology and expression of lineage markers over time. By virtue of this system, they demonstrated that SL-MkPs, which cannot be prospectively distinguished from other HSCs in terms of phenotypic markers or expression levels of megakaryocyte proteins, can efficiently induce expression of megakaryocyte protein such as CD41 in response to inflammatory signaling [polyinosinic:polycytidylic acid (pI:C) treatment], as well as cell types potentially capable of generating megakaryocytes (e.g., MkPs and MEPs), but unlike other subset of HSCs. Like HSCs, SL-MkPs are maintained in a highly potent and primitive state in homeostatic conditions. Upon inflammation, SL-MkPs are pushed toward maturation with increased megakaryocyte protein expression, and this state is distinct from MkPs, characterized by maintained high proliferative capacity by evading endomitosis, constituting an unique intermediate state between homeostatic SL-MkPs and MkPs. Intriguingly, inflammation-driven up-regulation of megakaryocyte proteins is not regulated at transcription level, but inflammation instructs efficient translation of megakaryocyte transcripts in HSCs. Single cell gene expression analysis revealed a small part of homeostatic LT-HSCs, as well as most of CD41high HSCs from pI:C treated mice, showed coordinated expression of megakaryocyte-related transcripts, with most transcripts being an “ON” state. These subsets can be clearly distinguished from other steady-state LT-HSCs or CD41low/med HSCs from pI:C treated mice, of which megakaryocyte transcript expression is regulated stochastically, with unique combinations of “ON” and “OFF” states of these transcripts depending on each cell. Importantly, overall transcriptome is otherwise quite similar between SL-MkPs and other HSCs. The authors postulated that this change from stochastic to coordinated megakaryocyte gene expression is associated with lineage commitment of HSCs to SL-MkPs. In spite of coordinated megakaryocyte transcript expression, SL-MkPs don’t translate these transcripts, and are mainly quiescent and metabolically inactive, sharing many features with HSCs rather than with MkPs. In response to acute inflammation, they are readily activated to produce megakaryocyte proteins from pre-existing megakaryocyte transcripts. Finally, the authors concluded SL-MkPs contribute little to steady-state megakaryopoiesis reflecting their quiescent nature, while they can serve as an emergency pool of platelets for inflammatory insult.
In this study, Haas et al. molecularly characterized unipotent SL-MkPs, as well as bypass from HSCs to MkPs (Figure 1), as was also indicated by previous studies (8,23). They identified distinct subsets within phenotypically HSCs based on both unilineage potential and coordinated expression patterns of megakaryocyte-related genes, respectively. Considering the subsets with coordinated megakaryocyte gene expression in steady-state conditions correspond to CD41high HSCs from pI:C treated mice in several aspects, the assumption that change in transcriptional program regulates lineage fate seems reasonable. Nevertheless, direct evidence that change from stochastic to coordinated megakaryocyte gene expression is accompanied by functional lineage commitment to SL-MkPs is lacking, because of retrospective nature of SL-MkP definition. The authors also argued that SL-MkPs contribute little to steady state megakaryopoiesis based on the fact that SL-MkPs are largely quiescent. Though transplantation remains the main experimental approach to assay differentiation potential of hematopoietic cells, transplanted cells have to undergo extraordinary stress during engraftment in distorted cytokine milieu, making it difficult to interpret results of fate mapping studies apart from hematopoietic stress (7). In this sense, most studies regarding megakaryopoiesis including those of Haas et al. were done in somewhat stressed conditions, and a role of SL-MkPs as well as cellular origins in non-stressed megakaryopoiesis remain to be clarified.
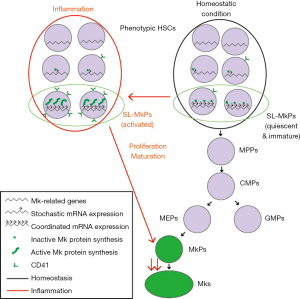
Haas et al. showed that only a small subfraction of vWF+ HSCs expressed all megakaryocyte transcripts and thus, SL-MkPs represent a megakaryocyte-committed subfraction of vWF+ HSCs. This finding is in accordance with the original work by Sanjuan-Pla et al. (14), as this study showed while some vWF+ HSCs expressed most of megakaryocyte-related genes assessed, typical vWF+ HSCs expressed only a part of them, in contrast to vWF− HSCs with little expression of these genes. Single cell transcriptome analysis by another group demonstrated that HSC subpopulation with homogeneous gene expression pattern and with higher HSC activity, showed higher expression of Vwf than the other subpopulation of HSCs (24). Importance of other megakaryocyte-related genes, however, was not underscored in this study, possibly reflecting a stochastic nature of expression of megakaryocyte-related genes in the most primitive HSCs. Collectively, findings from these studies suggest that most primitive HSCs tend to express at least some megakaryocyte-related genes, and coordinated megakaryocyte-related gene expression is associated with megakaryocyte-commitment rather than a primitive state. However, considering there remain substantial uncertainties regarding a relationship between megakaryocyte-biased subsets reported to date and functional implications of most megakaryocyte-related genes in stem cell maintenance, further studies are needed to elucidate the relation between the stemness and megakaryocyte-related gene expression.
The study by Haas et al. also demonstrated post-transcriptional megakaryocyte protein synthesis as a consequence of inflammation, at least when induced by pI:C and lipopolysaccharides (LPS). Of note are the facts that megakaryocyte protein synthesis is central in inflammation-driven megakaryocyte maturation and that they also shed light on the molecular basis of this post-transcriptional regulation. However, it remains to be determined to what extent the mechanisms are shared by different forms of inflammation, such as those of pI:C or LPS, both of which activate protein production depending on different pathways: STAT1- and mTOR-signaling for pI:C and TLR4/MYD88/TRIF-signaling for LPS, respectively. Furthermore, as inflammation is involved in pathogenesis of a long list of diseases, and platelets are increasingly appreciated for their association with inflammation (25), implication of SL-MkPs and megakaryocyte priming in thrombocytosis-associated diseases awaits further investigation.
Acknowledgements
Funding: This work was supported by in part by the Japan Society for the Promotion of Science (JSPS) KAKENHI (No. 15H01481 and No. 16K19567).
Footnote
Conflicts of Interest: The authors have no conflicts of interest to declare.
References
- Kondo M, Weissman IL, Akashi K. Identification of clonogenic common lymphoid progenitors in mouse bone marrow. Cell 1997;91:661-72. [Crossref] [PubMed]
- Akashi K, Traver D, Miyamoto T, et al. A clonogenic common myeloid progenitor that gives rise to all myeloid lineages. Nature 2000;404:193-7. [Crossref] [PubMed]
- Adolfsson J, Månsson R, Buza-Vidas N, et al. Identification of Flt3+ lympho-myeloid stem cells lacking erythro-megakaryocytic potential a revised road map for adult blood lineage commitment. Cell 2005;121:295-306. [Crossref] [PubMed]
- Forsberg EC, Serwold T, Kogan S, et al. New evidence supporting megakaryocyte-erythrocyte potential of flk2/flt3+ multipotent hematopoietic progenitors. Cell 2006;126:415-26. [Crossref] [PubMed]
- Boyer SW, Schroeder AV, Smith-Berdan S, et al. All hematopoietic cells develop from hematopoietic stem cells through Flk2/Flt3-positive progenitor cells. Cell Stem Cell 2011;9:64-73. [Crossref] [PubMed]
- Woolthuis CM, Park CY. Hematopoietic stem/progenitor cell commitment to the megakaryocyte lineage. Blood 2016;127:1242-8. [Crossref] [PubMed]
- Sun J, Ramos A, Chapman B, et al. Clonal dynamics of native haematopoiesis. Nature 2014;514:322-7. [Crossref] [PubMed]
- Yamamoto R, Morita Y, Ooehara J, et al. Clonal analysis unveils self-renewing lineage-restricted progenitors generated directly from hematopoietic stem cells. Cell 2013;154:1112-26. [Crossref] [PubMed]
- Yoshihara H, Arai F, Hosokawa K, et al. Thrombopoietin/MPL signaling regulates hematopoietic stem cell quiescence and interaction with the osteoblastic niche. Cell Stem Cell 2007;1:685-97. [Crossref] [PubMed]
- Kiel MJ, Yilmaz OH, Iwashita T, et al. SLAM family receptors distinguish hematopoietic stem and progenitor cells and reveal endothelial niches for stem cells. Cell 2005;121:1109-21. [Crossref] [PubMed]
- Novershtern N, Subramanian A, Lawton LN, et al. Densely interconnected transcriptional circuits control cell states in human hematopoiesis. Cell 2011;144:296-309. [Crossref] [PubMed]
- Månsson R, Hultquist A, Luc S, et al. Molecular evidence for hierarchical transcriptional lineage priming in fetal and adult stem cells and multipotent progenitors. Immunity 2007;26:407-19. [Crossref] [PubMed]
- Challen GA, Boles NC, Chambers SM, et al. Distinct hematopoietic stem cell subtypes are differentially regulated by TGF-beta1. Cell Stem Cell 2010;6:265-78. [Crossref] [PubMed]
- Sanjuan-Pla A, Macaulay IC, Jensen CT, et al. Platelet-biased stem cells reside at the apex of the haematopoietic stem-cell hierarchy. Nature 2013;502:232-6. [Crossref] [PubMed]
- Shin JY, Hu W, Naramura M, et al. High c-Kit expression identifies hematopoietic stem cells with impaired self-renewal and megakaryocytic bias. J Exp Med 2014;211:217-31. [Crossref] [PubMed]
- Zhao JL, Baltimore D. Regulation of stress-induced hematopoiesis. Curr Opin Hematol 2015;22:286-92. [PubMed]
- Essers MA, Offner S, Blanco-Bose WE, et al. IFNalpha activates dormant haematopoietic stem cells in vivo. Nature 2009;458:904-8. [Crossref] [PubMed]
- Mossadegh-Keller N, Sarrazin S, Kandalla PK, et al. M-CSF instructs myeloid lineage fate in single haematopoietic stem cells. Nature 2013;497:239-43. [Crossref] [PubMed]
- Ehninger A, Boch T, Uckelmann H, et al. Posttranscriptional regulation of c-Myc expression in adult murine HSCs during homeostasis and interferon-α-induced stress response. Blood 2014;123:3909-13. [Crossref] [PubMed]
- Cabezas-Wallscheid N, Klimmeck D, Hansson J, et al. Identification of regulatory networks in HSCs and their immediate progeny via integrated proteome, transcriptome, and DNA methylome analysis. Cell Stem Cell 2014;15:507-22. [Crossref] [PubMed]
- Muraoka K, Tsuji K, Yoshida M, et al. Thrombopoietin-independent effect of interferon-gamma on the proliferation of human megakaryocyte progenitors. Br J Haematol 1997;98:265-73. [Crossref] [PubMed]
- Haas S, Hansson J, Klimmeck D, et al. Inflammation-induced emergency megakaryopoiesis driven by hematopoietic stem cell-like megakaryocyte progenitors. Cell Stem Cell 2015;17:422-34. [Crossref] [PubMed]
- Nishikii H, Kanazawa Y, Umemoto T, et al. Unipotent megakaryopoietic pathway bridging hematopoietic stem cells and mature megakaryocytes. Stem Cells 2015;33:2196-207. [Crossref] [PubMed]
- Wilson NK, Kent DG, Buettner F, et al. Combined single-cell functional and gene expression analysis resolves heterogeneity within stem cell populations. Cell Stem Cell 2015;16:712-24. [Crossref] [PubMed]
- Semple JW, Italiano JE Jr, Freedman J. Platelets and the immune continuum. Nat Rev Immunol 2011;11:264-74. [Crossref] [PubMed]
Cite this article as: Masamoto Y, Kurokawa M. Inflammation-induced emergency megakaryopoiesis: inflammation paves the way for platelets. Stem Cell Investig 2016;3:16.