Sendai virus based direct cardiac reprogramming: what lies ahead?
Affecting over 38 million people worldwide, heart failure remains an immense challenge in health care (1). The pathology of heart failure involves death or dysfunction of cardiomyocytes and replacement with non-contractile scar tissue since the adult human heart lacks endogenous repair mechanisms to fully restore cardiac function after injury such as a myocardial infarction (MI). New therapeutic strategies for heart regeneration remains a top priority in the treatment of heart failure and may offer hope to this intractable disease. However, the ability to efficiently generate a large number of functioning cardiomyocytes capable of functional integration within the injured heart has remained a challenge in the field. Direct reprogramming of somatic cells such as fibroblasts to cardiomyocytes both in vitro and in vivo has recently emerged as a promising therapeutic candidate for treating heart failure.
Direct reprogramming was first reported in 1987 when cDNA encoding MyoD was transfected into fibroblasts and generated muscle myoblasts (2). The immense potential of this powerful tool for tissue regeneration and replacement was soon realized. There has been extensive focus on conversion of somatic cells to other lineages including myoblasts, neurons, hepatocytes, intestinal cells, blood progenitor cells, and cardiomyocytes (2-7). Direct reprogramming of fibroblasts into cardiomyocyte-like cells was first reported in 2010 by Ieda et al. where 14 cardiomyocyte-inducing factors were removed one by one in an iterative screening approach to identify those that were dispensable for direct reprogramming. This process ultimately identified three important cardiac developmental transcription factors (TFs), Gata4, Mef2c, and Tbx5 (GMT) sufficient to induce conversion of fibroblasts to cardiomyocyte-like cells without transitioning through a progenitor state (7). After the establishment of GMT as the core TFs for direct cardiac reprograming, much of the focus transitioned to improving the reprogramming efficiency and/or the function of the induced cardiomyocyte-like cells (iCMs) both in vitro and in vivo. Despite recent advances in this field, the majority of studies utilize integrating lentiviral or retroviral vectors for reprogramming, which could affect endogenous gene expression and are associated with the risk of insertional mutagenesis. Therefore, development of safer approaches to direct reprogramming is a necessity in the field to increase the translational ability of this approach.
In a recent paper by Miyamoto et al., the authors describe the use of integration-free non-pathogenic Sendai virus (SeV) vectors to express the cardiac reprogramming factors, GMT, in fibroblasts to generate iCMs both in vitro and in vivo (summarized in Figure 1) (8). In vitro reprogramming of mouse embryonic fibroblasts (MEFs) revealed that SeV reprogramming significantly increased both the efficiency and speed of reprogramming as compared to retrovirus transduction. SeV generated iCMs from these experiments showed stronger and earlier induction of cardiac genes, produced well-defined and organized sarcomeric structures and increased the percentage of beating cells. Functional analyses of SeV iCMs showed typical action potentials of all three cardiomyocyte subtypes (ventricular, atrial and nodal) and responses to neurohormonal stimulation similar to endogenous cardiomyocytes.
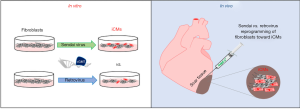
Next, the authors investigated the mechanism of why SeV reprogramming is faster and more efficient. Despite similar transduction efficiencies between SeV and retroviral constructs, the expression level of GMT was much higher in SeV treated MEFs and sustained for a longer period of time during reprogramming as compared to retrovirus treated MEFs. Additionally, SeV GMT expression, cardiac related gene expression and spontaneous beating could be controlled in a dose dependent manner while showing comparable GMT expression at a much lower MOI (1–5 vs. 20) than retroviral transduced cells. These findings suggested that robust and optimal GMT expression is the crucial factor in determining reprogramming efficiency and not other factors such as the SeV particles themselves or SeV induced iCMs proliferation.
Previous studies have highlighted that cardiac reprogramming in more differentiated fibroblasts such as mouse tail-tip fibroblasts (TTFs) or adult human cardiac fibroblasts (HCFs) is more difficult and require additional factors to increase efficiency (9-12). To test whether SeV vectors could also reprogram more mature fibroblasts Miyamoto et al. designed SeV vectors containing GMT plus Hand2 for mouse TTFs and GMT plus MESP1, MYOCD, and miR-133 for HCFs. Experiments similar to those performed in MEFS were carried out and showed that SeV vectors could generate functional iCMs while enhancing cardiac reprogramming efficiency over retroviral transduction in both TTFs and HCFs.
The ultimate goal of direct reprogramming is to be able to repair the damaged myocardium after injury in situ. Targeting endogenous cardiac fibroblasts through viral transduction in the infarct zone was attempted in 2012 by Song et al. and Qian et al., in which local delivery of GMT plus Hand2 retroviruses induced reprogramming of non-myocytes into iCMs by 4 weeks post-surgery (9,13). Interestingly, it has been reported that the in vivo cardiac niche may improve the efficiency of reprogramming, however, the mechanisms underlying this observation remain elusive and may be due to differences in experimental conditions (9,13-15).
Finally, to test the translational feasibility of SeV vectors for endogenous cardiac reprogramming, the authors performed in vivo cardiac reprogramming experiments using a mouse infarct model. Although SeV vectors have been shown to infect a myriad of cell types in vitro, the ability to infect specific cell types such as resident cardiac fibroblasts in vivo remain unknown. Injection of SeV containing GFP into infarcted mouse hearts showed that SeV primarily infected non-myocytes and fibroblasts similar to results seen using retrovirus vectors. Next, to test the reprogramming efficiency of SeV to iCMs in resident cardiac fibroblasts, a tamoxifen inducible fibroblast-lineage tracing model, Tcf21iCre/R26-tdTomato was used. After labeling TCF21-expressing cells using tamoxifen, permanent coronary artery ligation was performed and SeV GMT was directly injected in the peri-infarct region of the heart. One-week post injection about 1.5% of tdTomato+ cells expressed cTnT in SeV GMT injected animals whereas only 0.5% of tdTomato+ cells expressed cTnT in retrovirus treated animals. SeV GMT reprogrammed cells also exhibited clear cross striations after 1 week as compared to retrovirus reprogrammed cells which exhibited immature or no sarcomeric structures. Further experiments were carried out to evaluate the longer term continued reprogramming ability and functional recovery following MI in SeV GMT treated animals. Similar to retrovirus treatment, SeV GMT treatment was able to halt a further decrease in ejection fraction seen in control animals and reduce fibrosis in part by reducing collagen 1 protein expression in the scar area. Interestingly, SeV GMT significantly improved fractional shortening over both retrovirus and control treated animals after MI.
In summary, Miyamoto et al. show that SeV vectors expressing important cardiac TFs can be used to efficiently reprogram both mouse and human fibroblasts into integration-free iCMs in vitro. Additionally, SeV GMT was shown to improve in vivo direct cardiac reprogramming, maintain higher cardiac function and reduce scar size after MI compared with control animals. Although these data are promising and address a major obstacle in the field in overcoming the use of integrative viruses for reprogramming, several questions still remain. From the functional data presented by the authors, it appears that in vivo SeV reprogramming prevents the worsening of cardiac function after MI but does not improve cardiac function at four weeks post injury. As hypothesized by the authors, one potential mechanism for this observation is the reduction in collagen expression in the scar area. While reduction of collagen may offer an advantage following MI, another goal of direct cardiac reprogramming is to generate new cardiomyocytes in the scar area. Additionally, collagen production and scar formation post MI is an adaptive response to maintain ventricular wall strength and to prevent rupture. Long term studies are needed to determine whether transformation of fibroblasts immediately after an MI to iCMs will have an adverse effect on the ventricular wall durability and remodeling. This study evaluates the maturity of newly formed cardiomyocytes after reprogramming by immunostaining but does not address the functional integration of these cardiomyocytes into the heart using immunostaining for gap junction proteins and/or electrophysiological studies to determine whether iCMs electromechanically couple with the host myocytes. Evaluation of the integration and electrical properties of newly formed cardiomyocytes is an important consideration as introduction of focal arrythmias into the heart by direct reprogramming may prove fatal. Furthermore, it would also be of interest to evaluate the effectiveness of in vivo SeV reprogramming at time points beyond four weeks to address the long-term feasibility of this method in regard to survival and cardiac function.
Direct reprogramming technology offers great promise for cardiac regeneration therapy and this study addresses one important limitation that existed with this method. However, it is clear from this study and many others that the reprogramming process is very complex, and many factors have profound influence over its success. Several groups have reported improved programming efficiency in vivo compared to in vitro (9,13,14). These differences may be attributed to several factors including experimental conditions and fibroblast lineage tracing strategies. It is also possible that the heterogeneity of fibroblasts may affect their reprogramming, therefore further understanding of the epigenetic landscape of fibroblasts and their susceptibility to direct reprogramming would be of great use to the field. Continued research of key TFs, non-coding RNAs, small molecules, reprogramming mechanisms, delivery and targeting methods and biomaterials will help advance direct cardiac reprogramming to large animal models and ultimately for the treatment of heart failure. Furthermore, the refinement and improvement of SeV vectors will not only further progress in the cardiac reprogramming field, but other regenerative medicine fields as well.
Acknowledgements
Funding: R Ardehali is supported in part by the National Institute of Health DP2 (HL127728) and the California Institute for Regenerative Medicine (RN3-06378).
Footnote
Conflicts of Interest: The authors have no conflicts of interest to declare.
References
- Braunwald E. The war against heart failure: the Lancet lecture. Lancet 2015;385:812-24. [Crossref] [PubMed]
- Davis RL, Weintraub H, Lassar AB. Expression of a single transfected cDNA converts fibroblasts to myoblasts. Cell 1987;51:987-1000. [Crossref] [PubMed]
- Vierbuchen T, Ostermeier A, Pang ZP, et al. Direct conversion of fibroblasts to functional neurons by defined factors. Nature 2010;463:1035-41. [Crossref] [PubMed]
- Yu B, He ZY, You P, et al. Reprogramming fibroblasts into bipotential hepatic stem cells by defined factors. Cell Stem Cell 2013;13:328-40. [Crossref] [PubMed]
- Huang P, He Z, Ji S, et al. Induction of functional hepatocyte-like cells from mouse fibroblasts by defined factors. Nature 2011;475:386-9. [Crossref] [PubMed]
- Xie H, Ye M, Feng R, et al. Stepwise reprogramming of B cells into macrophages. Cell 2004;117:663-76. [Crossref] [PubMed]
- Ieda M, Fu JD, Delgado-Olguin P, et al. Direct reprogramming of fibroblasts into functional cardiomyocytes by defined factors. Cell 2010;142:375-86. [Crossref] [PubMed]
- Miyamoto K, Akiyama M, Tamura F, et al. Direct In Vivo Reprogramming with Sendai Virus Vectors Improves Cardiac Function after Myocardial Infarction. Cell Stem Cell 2018;22:91-103.e5. [Crossref] [PubMed]
- Song K, Nam YJ, Luo X, et al. Heart repair by reprogramming non-myocytes with cardiac transcription factors. Nature 2012;485:599-604. [Crossref] [PubMed]
- Yamakawa H, Muraoka N, Miyamoto K, et al. Fibroblast Growth Factors and Vascular Endothelial Growth Factor Promote Cardiac Reprogramming under Defined Conditions. Stem Cell Reports 2015;5:1128-42. [Crossref] [PubMed]
- Muraoka N, Yamakawa H, Miyamoto K, et al. MiR-133 promotes cardiac reprogramming by directly repressing Snai1 and silencing fibroblast signatures. EMBO J 2014;33:1565-81. [Crossref] [PubMed]
- Wada R, Muraoka N, Inagawa K, et al. Induction of human cardiomyocyte-like cells from fibroblasts by defined factors. Proc Natl Acad Sci U S A 2013;110:12667-72. [Crossref] [PubMed]
- Qian L, Huang Y, Spencer CI, et al. In vivo reprogramming of murine cardiac fibroblasts into induced cardiomyocytes. Nature 2012;485:593-8. [Crossref] [PubMed]
- Jayawardena TM, Finch EA, Zhang L, et al. MicroRNA induced cardiac reprogramming in vivo: evidence for mature cardiac myocytes and improved cardiac function. Circ Res 2015;116:418-24. [Crossref] [PubMed]
- Mohamed TM, Stone NR, Berry EC, et al. Chemical Enhancement of In Vitro and In Vivo Direct Cardiac Reprogramming. Circulation 2017;135:978-95. [Crossref] [PubMed]
Cite this article as: Engel JL, Ardehali R. Sendai virus based direct cardiac reprogramming: what lies ahead? Stem Cell Investig 2018;5:37.