Interleukin 8/KC enhances G-CSF induced hematopoietic stem/progenitor cell mobilization in Fancg deficient mice
Introduction
Fanconi anemia (FA) is a heterogeneous genetic disorder characterized by chromosomal instability, predisposition to malignancies, and progressive bone marrow failure which accounts for 90% of mortality in FA (1-7). Human leukocyte antigen identical allogeneic bone marrow transplantation from a family member is the most effective therapy for the treatment of FA patients with hematopoietic defects (8). Unfortunately, the availability of unaffected sibling donors is low for the majority of patients and the disease-free survival rate for transplants involving matched unrelated donors is suboptimal, ranging from 15% to 67% (9,10). However, despite past progress and the potential of new stem cell transplantation protocols to improve the outcomes, comparative analysis by Rosenberg et al. highlights an important and discouraging fact that greater than 50% of FA patients develop squamous cell carcinomas or other solid tumors by their mid-40s, perpetuated in part by hypersensitivity to DNA alkylating agents and irradiation in FA patients undergoing stem cell transplant (11). Thus, new interventions are needed to reduce the incidence of squamous cell carcinomas in FA patients who receive transplants (12-14). In addition, FA patients have higher rates of graft failure, graft-versus-host disease, and infections, leading to significantly increased rates of morbidity and mortality (8). Gene therapy has been proposed as a strategy for improving treatment outcomes in FA associated bone marrow failure, while preventing leukemia and reducing the risk for subsequent squamous cell carcinomas. The potential benefit of hematopoietic cell gene therapy, even if a relatively low frequency of hematopoietic stem/progenitor cells (HSPCs) express the wild-type gene product, is supported by the observations that some individuals with FA develop self-correcting mutations in one of the FA genes, resulting in a single corrected stem cell that is capable of repopulating the bone marrow and restoring physiological hematopoiesis (15).
Mobilization of autologous stem cells from the bone marrow into the peripheral blood (PB) for harvesting has been thought to be ideal prior to the onset of bone marrow failure, which often occurs during childhood. Granulocyte colony-stimulating factor (G-CSF) is a US Food and Drug Administration (FDA)—approved therapy for mobilization of HSPCs for autologous transplantation in a variety of clinical settings (16). However, patients with FA demonstrate a markedly decreased response to G-CSF (17). Thus, an alternative approach to improve HSPC collection in FA patients represents a significant unmet clinical need. Recently, our laboratory and others have reported a synergetic effect between G-CSF and AMD3100 or NSC23766 to mobilize repopulating stem cells in FA knockout mice (18,19).
Interleukin-8 (IL-8, also known as KC in rodents) is a chemoattractant that induces rapid mobilization of HSPCs capable of repopulating host hematopoietic tissues completely and permanently (20,21). In the present study, we report that KC levels are markedly reduced in the serum of Fancg deficient (Fancg–/–) mice due to deficient KC secretion by mesenchymal stem/progenitor cells (MSPCs). Analogous to FA patients, HSPC mobilization in response to G-CSF is significantly diminished in Fancg–/– mice. Here, we demonstrate that combinatorial administration of KC and G-CSF is sufficient to significantly increase HSPC mobilization in Fancg–/– mice. These results demonstrate that KC in combination with G-CSF can overcome the mobilization failure in a murine model of FA, thus providing key pre-clinical evidence supporting the utility of G-CSF plus IL-8 to collect sufficient numbers of HSPCs from FA patients undergoing gene therapy.
Materials and methods
Animals and reagents
Fancg heterozygous (Fancg+/–) mice utilized in these studies have been previously described (22). Fancg+/– (C57Bl/6 × SV129) mice were backcrossed into a C57Bl/6J strain and then bred to generate Fancg–/– and WT mice. All protocols were approved by the Institutional Animal Care and Use Committee at Indiana University School of Medicine. Six- to eight-week-old mice were used in these studies. Murine G-CSF, KC, murine recombinant granulocyte-macrophage colony-stimulating factor (GM-CSF), murine interleukin-3 (IL-3) and murine stem cell factor (SCF) were purchased from PeproTech (Rocky Hill, NJ, USA), macrophage colony-stimulating factor (M-CSF) was purchased from R&D Systems (Minneapolis, MN, USA). Antibodies for flow cytometry were purchased from BD-Pharmingen (San Diego, CA, USA) unless otherwise stated.
MSPC culture and preparation of conditioned media (CM)
MSPCs were generated as previously described (23). Briefly, bone marrow mononuclear cells (BMMNCs) were isolated from age-and gender-matched WT and Fancg–/– mice and MSPC cultures were established. MPSCs at passages 7-10 were used in the following experiments. In order to obtain the CM, 1×106 MSPCs were plated in 10cm dishes and expanded. After reaching 80% confluence, culture media was changed to media without fetal bovine serum (FBS) and the MSPCs were starved for 24 hours. The CM and MSPCs were then collected for further experiments.
Purification of HSPCs from murine bone marrow
HSPCs were purified as described previously (24). Briefly, BMMNCs were enriched by immune-magnetic lineage negative (Lin–) selection (MACS; Miltenyi Biotech, Auburn, CA, USA) with the use of a mixture of purified rat anti-mouse mAbs specific for the mature cell lineage antigens CD45R (B220, Clone RA3-6B2), Gr-1 (Ly-6G, Clone RB6-8C5), CD4 (L3T4, Clone RM4-5), CD8a (Ly-2, Clone 53-6.7), TER119 (TER119), and Mac-1 (CD11b, Clone M1/70). The nonmagnetic Lin– fraction was collected and incubated with rat anti-mouse CD32/CD16 to avoid nonspecific antibody binding and then stained with fluoresceinated (FITC) rat anti-mouse CD117 (c-Kit). Negative control cells were stained with FITC-conjugated IgG2b. Based on these controls, Lin–c-Kit+ cells were isolated by sorting with a fluorescence-activated cell sorter (FACStar Plus; Becton Dickinson) under sterile conditions. The purity of Lin–c-Kit+ cells obtained was >90%.
Migration assays of HSPCs
Lin–c-Kit+ cells (2×104/well) in 100 μL DMEM were added into the upper well of 24-transwell with 5 µm pore size (Costar, Acton, MA, USA). The CM collected from MSPCs was loaded into the lower wells in 500 µL volume and incubated at 37 °C, 5% CO2. After 12 hours, the cells migrating into the lower wells were harvested and counted. The migration rate was calculated as the percentage of the number of cells migrating into the lower wells divided by the total number of cells loaded.
Detection of cytokines in CM and serum
To measure MSPC cytokine production, MSPC CM was subjected to protein array analysis with RayBio mouse cytokine antibody array II & 2.1 (Raybiotech Inc., Norcross, USA) as described previously (25). Enzyme-linked immunosorbent assays (ELISA, Mouse CXCL1/KC Quantikine ELISA Kit, R&D Systems) were conducted to quantitatively measure the concentration of altered growth factor(s) identified from the protein array analysis according to the manufacturer’s instructions.
HSPC mobilization and colonogenic assays
To examine the effect of KC and G-CSF on HSPC mobilization, 1,500 µg/kg of G-CSF, with or without KC (1,500 μg/kg), was delivered by intraperitoneal injection to Fancg–/– mice and syngeneic controls for 5 days. The final injection was administered 1 hour before the mice were euthanized. PB and spleens were harvested and MNCs were then separated by low-density gradient centrifugation. Cellularity and GM colony-forming units (CFU-GM) were evaluated as previously described (26). Briefly, 5×104 MNCs were seeded onto a 35-mm gridded dish in medium containing methylcellulose and murine GM-CSF 10 ng/mL, IL-3 5 ng/mL, SCF 50 ng/mL and M-CSF 10 ng/mL. After incubation at 37 °C, 5% CO2 for 7 days, the number of CFU-GM was scored and analyzed.
Statistical analysis
Student’s t-test and one-way ANOVA were used to evaluate statistical differences between WT and Fancg–/– cells. Statistical significance was defined as P values less than 0.05.
Results
Reduced KC production in serum and MSPC CM of Fancg–/– mice
MSPCs have been shown to release a number of cytokines/growth factors impacting hematopoiesis (27). To elucidate whether Fancg deficiency alters MSPC cytokine production, we performed protein array analysis using CM collected from WT or Fancg–/– MSPCs as described previously (26,28). While there was equivalent protein loading, multiple growth factors including MCP-1, KC (equivalent to IL-8 in humans), IL-6, and RANTES were found to be downregulated in Fancg–/– MSPC CM (Figure 1A). Since KC is involved in chemotaxis and has ability to promote rapid mobilization of HSPCs (20,21). We further analyzed the effects of impaired KC production in the following experiments.
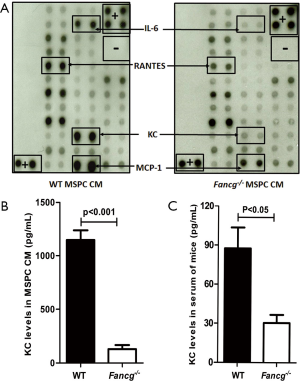
Quantitative measurement of KC levels by ELISA confirmed its presence in significantly reduced levels in Fancg–/– MSPC CM as compared to WT controls (Figure 1B). Given that KC is produced by not only stromal cells but also endothelial cells and monocytes, we further assessed KC levels in murine serum. Consistently, we observed a greater than two-fold reduction in KC levels in the serum of Fancg–/– mice compared with WT serum (Figure 1C).
Reduced HSPC chemoattractive capacity of Fancg–/– MSPC CM
Utilizing a genetic murine model of FA, we previously demonstrated that Fancg–/– MSPCs exhibit marked defects in proliferation, survival, and hematopoietic supportive activity (26). In the present study, we sought to further examine whether the capacity of Fancg–/– MSPCs supernatants to promote HSPC migration was different from that of WT controls. As shown in Figure 2, the migration rate of both WT and Fancg–/– HSPCs was significantly reduced in response to Fancg–/– MSPC CM compared to WT controls. Based on these findings, we reasoned that certain chemoattractants hyposecreted by Fancg–/– MSPCs may play a critical role in this impaired migratory capacity of HSPCs.
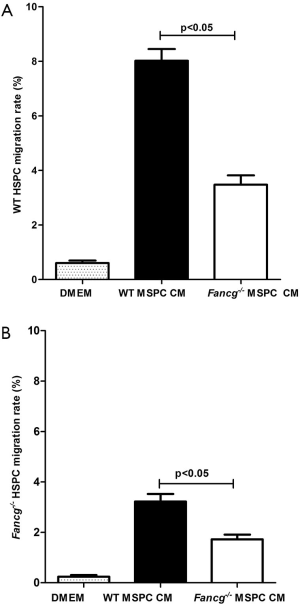
KC levels in MSPC CM pivotally impact HSPC migration
To further test whether KC levels in MSPC CM may be a critical factor influencing the migratory response of HSPCs, we repeated the migration assays described above with addition of either exogenous KC or KC antibody to the MSPC CM. As expected, addition of KC antibody (20 μg/mL) to WT MSPC CM significantly reduced the migration rate of WT HSPCs, from 6% to 1%, a level equivalent to that of the DMEM negative control (Figure 3A). In contrast, addition of KC (100 ng/mL) to Fancg–/– MSPC CM significantly increased the migratory response of both WT and Fancg–/– HSPCs (Figure 3B). Collectively, these data indicate a pivotal role for KC as the dominant MSPC secreted factor promoting HSPC migration.
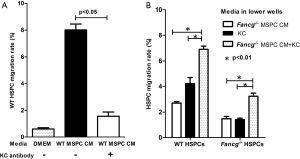
Co-administration of G-CSF and KC enhances HSPC mobilization in vivo
It has been reported that patients with FA exhibit a markedly decreased response to G-CSF induced stem cell mobilization and that endogenous IL-8 production is critical for this process (17,29). We therefore sought to evaluate whether deficient KC/IL-8 production by Fancg–/– MSPCs may be a pivotal factor hindering the efficacy of G-CSF mediated hematopoietic stem cell mobilization in vivo in a Fancg–/– murine model of FA. Analogous to FA patients, HSPC mobilization in response to G-CSF is significantly diminished in Fancg–/– mice, whereby G-CSF administration significantly increased the number of CFU-GM in PB of WT mice, but with no observable effect in Fancg–/– mice (Figure 4A). By contrast, however, we demonstrate that co-injection of G-CSF with KC significantly increased CFU-GM numbers not only in WT mice but also in Fancg–/– mice. Moreover, we observed a synergetic effect of combinatorial G-CSF plus KC therapy in Fancg–/– mice, whereby the CFU-GM number increased by 2.5-fold in Fancg–/– mice, as opposed to only a 1.3-fold increase in WT mice versus G-CSF monotherapy (Figure 4A). The number of CFU-GM obtained from spleens of Fancg–/– and WT mice demonstrated similar results (Figure 4B).
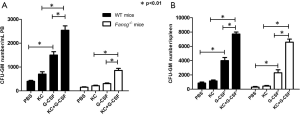
Discussion
A potential therapeutic option for treating FA dependent bone marrow failure is to collect mobilized HSPCs from PB prior to the development of severe pancytopenia, and infuse them when symptomatic bone marrow failure occurs. Unfortunately, the markedly decreasing mobilization of HSPCs in response to G-CSF in FA patients represents a significant hindrance to the utility of this approach (17). We demonstrate here that co-injection of G-CSF and KC can synergistically induce HSPC mobilization into circulating blood in a murine model of FA, suggesting a potential clinical application of combined G-CSF and IL-8/KC administration in facilitating HSPC mobilization in FA patients prior to the onset of bone marrow failure.
KC, the rodent equivalent of human IL-8, is produced by many cells, including monocytes, fibroblasts and endothelial cells. Laterveer et al. have demonstrated that administration of a single dose of KC can significantly increase circulating CFU-GM between 5 and 15 minutes following injection (20,21). In our study, KC alone was insufficient to increase circulating CFU-GM, a discrepancy which may relate to differing experimental approaches by which KC was injected over a 5-day timecourse with analyses conducted 60 minutes after the final injection, as opposed to 5 to 15 minutes following a single injection of KC in studies by Laterveer et al. (20,21). Nonetheless, we demonstrate that KC co-injection with G-CSF can synergistically increase CFU-GM numbers in both PB and spleens of Fancg–/– mice, which was unable to accomplish by either G-CSF or KC administration alone.
It is widely accepted that MSPCs express/secrete cytokines, extracellular matrix proteins and cell adhesion molecules and support the homing, migration, proliferation and survival of HSPCs (30,31). In addition to our previously described functional deficits in Fancg–/– MSPCs (26), here we demonstrate that several secreted proteins, including MCP-1, KC, IL-6, and RANTEs are downregulated in Fancg–/– MSPC CM. It has been reported that impaired production of stem cell factor, stromal cell-derived factor-1 and vascular cell adhesion molecule-1 within the bone marrow niche play a key role in HSPC mobilization by G-CSF (32-34). Intriguingly, however, none of these factors were found to be misexpressed in our study, thereby excluding their role in the impaired migration of HSPCs in response to Fancg–/– MSPC CM. Though there is no obvious evidence show that MCP-1, IL-6 and RANTEs were involved in mobilization of HSPCs in FA patients, their biological functions of downregulation in Fancg–/– MSPC CM need to be elucidated in further study.
Two pharmacologic agents, AMD3100 and NSC23766 have been reported to synergize with G-CSF in mobilization of HSPCs in Fanca–/– mice (18,19). Compared to these compounds, KC/IL-8 functions as a chemoattractant produced endogenously by monocytes, stromal and endothelial cells and thus may have less safety issues than synthetic agents. To-date, many cytokines or chemoattractants have been reported to be altered in patients with FA (35-38). However, whether serum IL-8 levels are reduced universally in FA patients versus only in certain subtypes of FA needs to be further investigated.
The microenvironment, also known as niche, has been shown to play a critical role in sustaining hematopoietic stem cell functions and modulating stem cell differentiation. The microenvironment represents a defined anatomical compartment that provides regulatory signals to HSPCs via interactions with the microenvironment through a three-dimensional matrix of cells, extracellular matrix molecules, and cell-bound or soluble cytokines and chemokines (39-42). MSPCs in the microenvironment have been shown to express/secrete cytokines, extracellular matrix proteins and cell adhesion molecules which support the homing, migration, proliferation and survival of HSPCs (30,31). Utilizing a genetic murine model of FA, Fancg–/– mice, we recently showed that Fancg–/– MSPCs exhibit marked defects in proliferation, survival, and hematopoietic supportive activity both in vivo and in vitro (26). While alterations in cytokine production by human FA fibroblasts were first reported by several groups nearly two decades ago, limitations in co-culture experiments have led to controversial conclusions (6,7,43). To-date, little information is available on the relationship between altered HSPC mobilization and mesenchymal lineage defects in FA. In summary, our results suggest that KC/IL-8 could be proved useful in the synergistic mobilization of FA HSPCs in combination with G-CSF.
Acknowledgements
We thank Heather Daniel for administrative support.
Funding: This work was supported in part by the National Natural Science Foundation of China (No. 81270575, No. 81330015), Natural Science Foundation of Tianjin (No. 11JCYBJC10500), the Leukemia Lymphoma Society (LLS 6234-12 to FCY), and NIH R01 CA155294-01 (to DWC, HH, and FCY).
Footnote
Conflicts of Interest: The authors have no conflicts of interest to declare.
References
- Auerbach AD, Buchwald M, Joenje H. Fanconi anemia. In: Vogelstein B, Kinzler KW. eds. The Genetic Bases of Human Cancer, 2nd ed. New York: McGraw-Hill, 2002.
- Mathew CG. Fanconi anaemia genes and susceptibility to cancer. Oncogene. 2006;25:5875-84. [PubMed]
- Bagby GC, Alter BP. Fanconi anemia. Semin Hematol 2006;43:147-56. [PubMed]
- Smogorzewska A, Matsuoka S, Vinciguerra P, et al. Identification of the FANCI protein, a monoubiquitinated FANCD2 paralog required for DNA repair. Cell 2007;129:289-301. [PubMed]
- Kook H. Fanconi anemia: current management. Hematology 2005;10:108-10. [PubMed]
- Dilloo D, Vöhringer R, Josting A, et al. Bone marrow fibroblasts from children with aplastic anemia exhibit reduced interleukin-6 production in response to cytokines and viral challenge. Pediatr Res 1995;38:716-21. [PubMed]
- Bagby GC Jr, Segal GM, Auerbach AD, et al. Constitutive and induced expression of hematopoietic growth factor genes by fibroblasts from children with Fanconi anemia. Exp Hematol 1993;21:1419-26. [PubMed]
- MacMillan ML, Hughes MR, Agarwal S, et al. Cellular therapy for fanconi anemia: the past, present, and future. Biol Blood Marrow Transplant 2011;17:S109-14. [PubMed]
- Wagner JE, Eapen M, MacMillan ML, et al. Unrelated donor bone marrow transplantation for the treatment of Fanconi anemia. Blood 2007;109:2256-62. [PubMed]
- Yabe M, Yabe H, Matsuda M, et al. Bone marrow transplantation for Fanconi anemia. Adjustment of the dose of cyclophosphamide for preconditioning. Am J Pediatr Hematol Oncol 1993;15:377-82. [PubMed]
- Rosenberg PS, Socié G, Alter BP, et al. Risk of head and neck squamous cell cancer and death in patients with Fanconi anemia who did and did not receive transplants. Blood 2005;105:67-73. [PubMed]
- Berger R, Bernheim A, Gluckman E, et al. In vitro effect of cyclophosphamide metabolites on chromosomes of Fanconi anaemia patients. Br J Haematol 1980;45:565-8. [PubMed]
- Auerbach AD, Adler B, O’Reilly RJ, et al. Effect of procarbazine and cyclophosphamide on chromosome breakage in Fanconi anemia cells: relevance to bone marrow transplantation. Cancer Genet Cytogenet 1983;9:25-36. [PubMed]
- Gluckman E, Devergie A, Dutreix J. Radiosensitivity in Fanconi anaemia: application to the conditioning regimen for bone marrow transplantation. Br J Haematol 1983;54:431-40. [PubMed]
- Tolar J, Adair JE, Antoniou M, et al. Stem cell gene therapy for fanconi anemia: report from the 1st international Fanconi anemia gene therapy working group meeting. Mol Ther 2011;19:1193-8. [PubMed]
- Möhle R, Kanz L. Hematopoietic growth factors for hematopoietic stem cell mobilization and expansion. Semin Hematol 2007;44:193-202. [PubMed]
- Croop JM, Cooper R, Fernandez C, et al. Mobilization and collection of peripheral blood CD34+ cells from patients with Fanconi anemia. Blood 2001;98:2917-21. [PubMed]
- Pulliam AC, Hobson MJ, Ciccone SL, et al. AMD3100 synergizes with G-CSF to mobilize repopulating stem cells in Fanconi anemia knockout mice. Exp Hematol 2008;36:1084-90. [PubMed]
- Milsom MD, Lee AW, Zheng Y, et al. Fanca–/– hematopoietic stem cells demonstrate a mobilization defect which can be overcome by administration of the Rac inhibitor NSC23766. Haematologica 2009;94:1011-5. [PubMed]
- Laterveer L, Lindley IJ, Heemskerk DP, et al. Rapid mobilization of hematopoietic progenitor cells in rhesus monkeys by a single intravenous injection of interleukin-8. Blood 1996;87:781-8. [PubMed]
- Laterveer L, Lindley IJ, Hamilton MS, et al. Interleukin-8 induces rapid mobilization of hematopoietic stem cells with radioprotective capacity and long-term myelolymphoid repopulating ability. Blood 1995;85:2269-75. [PubMed]
- Si Y, Ciccone S, Yang FC, et al. Continuous in vivo infusion of interferon-gamma (IFN-gamma) enhances engraftment of syngeneic wild-type cells in Fanca–/– and Fancg–/– mice. Blood 2006;108:4283-7. [PubMed]
- Wu X, Estwick SA, Chen S, et al. Neurofibromin plays a critical role in modulating osteoblast differentiation of mesenchymal stem/progenitor cells. Hum Mol Genet 2006;15:2837-45. [PubMed]
- Yang FC, Atkinson SJ, Gu Y, et al. Rac and Cdc42 GTPases control hematopoietic stem cell shape, adhesion, migration, and mobilization. Proc Natl Acad Sci U S A 2001;98:5614-8. [PubMed]
- Yang FC, Ingram DA, Chen S, et al. Neurofibromin-deficient Schwann cells secrete a potent migratory stimulus for Nf1+/– mast cells. J Clin Invest 2003;112:1851-61. [PubMed]
- Li Y, Chen S, Yuan J, et al. Mesenchymal stem/progenitor cells promote the reconstitution of exogenous hematopoietic stem cells in Fancg–/– mice in vivo. Blood 2009;113:2342-51. [PubMed]
- Stavroulaki E, Kastrinaki MC, Pontikoglou C, et al. Mesenchymal stem cells contribute to the abnormal bone marrow microenvironment in patients with chronic idiopathic neutropenia by overproduction of transforming growth factor-β1. Stem Cells Dev 2011;20:1309-18. [PubMed]
- Yang FC, Chen S, Robling AG, et al. Hyperactivation of p21ras and PI3K cooperate to alter murine and human neurofibromatosis type 1-haploinsufficient osteoclast functions. J Clin Invest 2006;116:2880-91. [PubMed]
- Watanabe T, Kawano Y, Kanamaru S, et al. Endogenous interleukin-8 (IL-8) surge in granulocyte colony-stimulating factor-induced peripheral blood stem cell mobilization. Blood 1999;93:1157-63. [PubMed]
- Honczarenko M, Le Y, Swierkowski M, et al. Human bone marrow stromal cells express a distinct set of biologically functional chemokine receptors. Stem Cells 2006;24:1030-41. [PubMed]
- Kassem M. Mesenchymal stem cells: biological characteristics and potential clinical applications. Cloning Stem Cells 2004;6:369-74. [PubMed]
- Semerad CL, Christopher MJ, Liu F, et al. G-CSF potently inhibits osteoblast activity and CXCL12 mRNA expression in the bone marrow. Blood 2005;106:3020-7. [PubMed]
- Christopher MJ, Link DC. Granulocyte colony-stimulating factor induces osteoblast apoptosis and inhibits osteoblast differentiation. J Bone Miner Res 2008;23:1765-74. [PubMed]
- Singh P, Hu P, Hoggatt J, et al. Expansion of bone marrow neutrophils following G-CSF administration in mice results in osteolineage cell apoptosis and mobilization of hematopoietic stem and progenitor cells. Leukemia 2012;26:2375-83. [PubMed]
- Dufour C, Corcione A, Svahn J, et al. TNF-alpha and IFN-gamma are overexpressed in the bone marrow of Fanconi anemia patients and TNF-alpha suppresses erythropoiesis in vitro. Blood 2003;102:2053-9. [PubMed]
- Briot D, Macé-Aimé G, Subra F, et al. Aberrant activation of stress-response pathways leads to TNF-alpha oversecretion in Fanconi anemia. Blood 2008;111:1913-23. [PubMed]
- Ibáñez A, Río P, Casado JA, et al. Elevated levels of IL-1beta in Fanconi anaemia group A patients due to a constitutively active phosphoinositide 3-kinase-Akt pathway are capable of promoting tumour cell proliferation. Biochem J 2009;422:161-70. [PubMed]
- Justo GA, Bitencourt MA, Pasquini R, et al. Increased IL10 plasmatic levels in Fanconi anemia patients. Cytokine 2013;64:486-9. [PubMed]
- Li Z, Li L. Understanding hematopoietic stem-cell microenvironments. Trends Biochem Sci 2006;31:589-95. [PubMed]
- Ho AD, Wagner W. The beauty of asymmetry: asymmetric divisions and self-renewal in the haematopoietic system. Curr Opin Hematol 2007;14:330-6. [PubMed]
- Krause DS. Plasticity of marrow-derived stem cells. Gene Ther 2002;9:754-8. [PubMed]
- Raaijmakers MH, Scadden DT. Evolving concepts on the microenvironmental niche for hematopoietic stem cells. Curr Opin Hematol 2008;15:301-6. [PubMed]
- Stark R, Andre C, Thierry D, et al. The expression of cytokine and cytokine receptor genes in long-term bone marrow culture in congenital and acquired bone marrow hypoplasias. Br J Haematol 1993;83:560-6. [PubMed]
Cite this article as: Li Y, Xing W, He YZ, Chen S, Rhodes SD, Yuan J, Zhou Y, Shi J, Bai J, Zhang FK, Yuan WP, Cheng T, Xu MJ, Yang FC. Interleukin 8/KC enhances G-CSF induced hematopoietic stem/progenitor cell mobilization in Fancg deficient mice. Stem Cell Investig 2014;1:19.